Revisão
Structure-activity relationship of triterpenoid saponins: Biological properties and commercial applicabilities
Abstract
Saponins are plant-derived non-ionic surfactants and widely applied in many products, such as cosmetics, cleansers, medicines, vaccine, as additives by therapeutic properties and chemical characteristics theirs. These substances are of growing interest for drug research as they're active constituents of several folk medicines, in addition to comprising an important class of medicinal chemistry. Triterpenoid saponins are secondary metabolites, these're largely distributed in plant species and they're characterized as one of the active principles of these. These saponins have potent anti-viral, adjuvant, hemolytic, cytotoxic and anti-angiogenic activities that are relationship with the presence of characteristics chemical moiety. In this work was summarized the studies found in the scientific literature on the therapeutic properties of triterpenoid saponins, as well as commercial applicability theirs. Recent works have suggested some triterpenoid saponins like candidates for treatment of patients with COVID-19.
- Keywords:
- Triterpenoid saponins.
- Medicinal chemistry.
- Therapeutics properties.
- Vaccine.
- COVID-19.
Introduction
Saponins are secondary metabolites derived from many edible and inedible parts of plants, such as roots, bark, stems, leaves, fruits and seeds. They're natural non-ionic surfactants formed by at least one polar and nonpolar chemical moiety[1-3]. Beyond that, they could form stable foam like soap in aqueous solutions[4]. A preliminary test usually used to detect the presence of saponins in natural products, is the addition of water on the plant material in the proportion of 5:1, followed by constant agitation for one minute. The FIGURE 1 shows a saponin detection test using the barks of the species Ziziphus joazeiro Mart. commonly used as a natural surfactant in products for cosmetic and cleaner use due to the presence of hydrophilic and hydrophobic portions characteristic of triterpenic saponins[5].
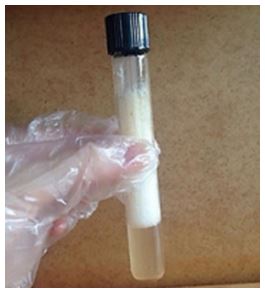
The pure saponins are white, non-volatile, and highly hygroscopic columnar crystal. It's soluble in alkaline aqueous solution, hot glacial acetic acid, methanol, ethanol, petroleum ether and other solvents. Triterpedoid saponins are most common than steroidal saponins and occur mainly in the dicotyledonous angiosperms. Triterpenoid saponins consist of a triterpenoid aglycone, which consists of a C30 skeleton, comprising a tetracyclic or pentacyclic sapogenin.
The pentacyclic triterpenes can be divided into three main groups, according to their skeleton: β-amirina or oleananos which presents two methyl groups in C-20. Those of the type α-amirina or bears that present a methyl group in C-20 and another one in C-19. The stereochemistry in this saponins between rings A/B, B/C and C/D are cis. The lupeol-type saponins differ from those previously mentioned in the stereochemistry between D/E rings, which is trans. In addition, the fifth ring (E) has 5 carbons, not being hexagonal as in other triterpene saponins (FIGURE 2).
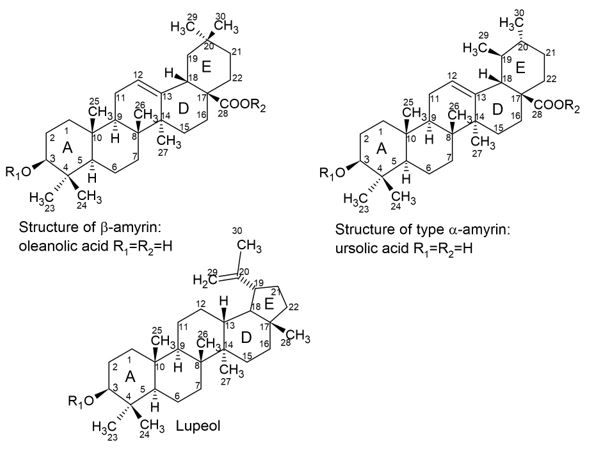
Substituents in the basic nucleus, such as hydroxyls, carboxyls and methoxyls, differentiate the compounds identified in this group. Carbohydrates are generally linked as an ether bond at C-3 or at the carboxyl at C-28 as an ester bond. When double bond occurs, it is present between C-12 and C-13 in oleanans, ursans and lupeol groups. Other types of nucleus more rarely found are friedelano, taraxastano and hopano[5].
Triterpenoid saponins may also have monoterpenes (C10) attached to their structure. These monoterpenic portions are responsible for the designation of complex saponins. Both triterpenoid and complex triterpenoid saponins stand out for being bioactive and continue as a source of research for obtaining drugs (FIGURE 3)[6].
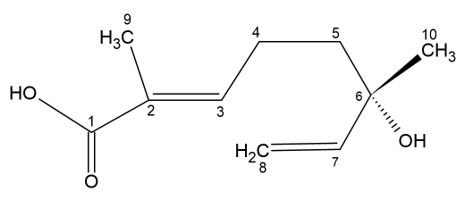
There are many commercial applications for triterpenoid saponins. These substances have been used such as cosmetics, cleansers, vaccines and medicines as additives by therapeutic properties and detergent action theirs. Triterpenoid saponins have been shown to be promising in the treatment of several pathologies and the applicability of these secondary metabolites is directly related to its chemical structure that gives the characteristic of surfactants. Surfactants are typically organic compounds containing hydrophobic and hydrophilic groups bearing polar and non-polar classes. Thus, saponins stand out not only for their cleaning power, but also for promoting health benefits for animals and human beings, whose attributions are directly related to chemical groups of these substances[7,8].
For a better understanding of this class of substances it is necessary to talk about its biosynthesis, as well as to understand the difference between primary and secondary metabolites.
The plants and microorganisms produce an immeasurable amount of compounds with highly complex chemical structures that don't have well-defined functions for producing organisms, since they don't fulfill a certain role in metabolism, growth and cell division. These low molecular weight metabolites are called special metabolites or secondary metabolites and may have potential therapeutic relevance[5].
Secondary metabolites can be opposed to products of the basal metabolism, called primary metabolites. As an example of the primary metabolites are amino acids, sugars, nucleotides, vitamins and fatty acids. Thus, basal metabolism includes all compounds and processes that are essential for an individual's growth and development and is characterized by being universally similar[5].
All special metabolites are derived from components of basal metabolism. Thus, the biosynthesis of several special metabolites can be derived from three basic principles: the rules of isoprene (C-5), acetate and amino acids. There are two possible biosynthetic pathways for the formation of saponins: the mevalonate pathway and the deoxy xylulose phosphate pathway[9].
Material and Methods
In this review are summarized the studies found in the scientific literature about Structure-activity relationship of triterpenoid saponins, biological properties and commercial applicabilities in the last two decades. The main scientific bases used for the development of this work were Science Direct, Capes periodicals portal and Google academic.
Results and Discussion
The search in Science Direct, Capes periodicals portal and Google academic using the terms "Triterpenoid saponins" resulted in 7,452; 6,945 and 33,600 articles respectively. This large amount of articles show that research on triterpenoid saponins is of great relevance, arousing the interest primarily of researchers in the field of medicinal chemistry and health. The following topics will address the two biosynthetic pathways described in the literature (FIGURE 4 and FIGURE 5), the biological properties and activity-structure relationship. Finally, will be discussed the Commercial applicability of triterpenoidal saponins that was summarized in the TABLE 3.
Biosynthesis of triterpenoid saponins
Initially the mevalonate pathway involves 3R-mevalonic acid as intermediate, with the formation of two isoprene units (C5) like the 3,3'-dimethylalyl pyrophosphate (DMAPP) and 3-isopentenyl pyrophosphate (IPP). The head-tail condensation reaction between these two isoprene units (2-methyl-butadiene), catalyzed by the enzyme prenyltransferase, forms the chain of geranyl pyrophosphate (GPP), precursor to monoterpenes (C10) [9]. From the union between isopentenyl pyrophosphate (IPP) and pyrophosphategeranyl (GPP) farnesyl pyrophosphate (FPP) is obtained and the condensation between these two C15 units form chains of 30 carbon atoms, a precursor to triterpenes. The carbonaceous precursor chain of triterpenes is squalene, which is oxidized to squalene oxide, being the common starting point for cyclization reactions in the triterpenoid biosynthesis (FIGURE 4) while carbohydrates originate from primary metabolism and are added later to a triterpene nucleus interspersed with monoterpenes for complex triterpenoid saponins[2].
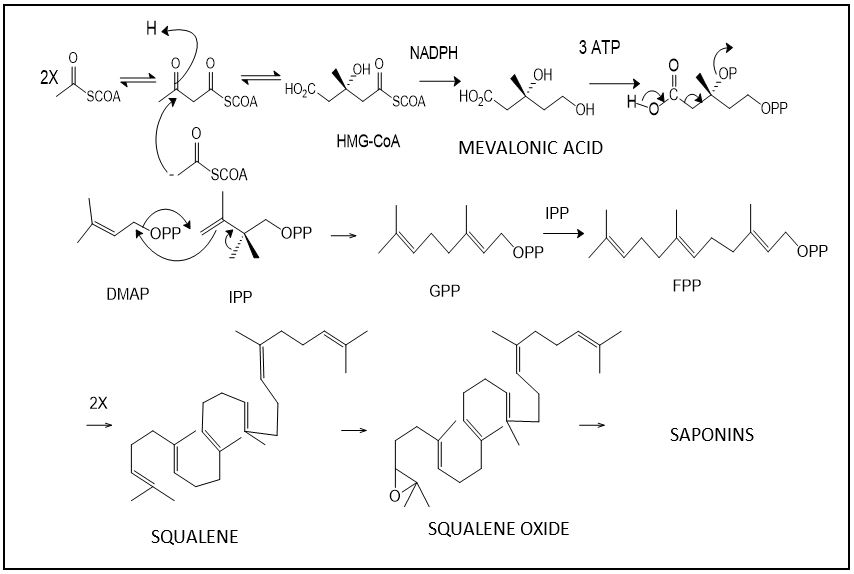
The deoxy xylulose phosphate pathway was discovered more recently and is believed to be more widespread in nature than the mevalonate pathway. The 1-deoxy-D-xylulose-5-phosphate is the chemical intermediate involved in this biosynthetic pathway and is formed from two products of glycolysis, pyruvic acid and D-glyceraldehyde-3P, in a process mediated by the coenzyme thiamine diphosphate (TPP)[5].
Through a pinacol-pinacolone rearrangement and followed by reduction, the deoxy xylulose phosphate is converted to 2-methyl-D-erythritol-4-phosphate, giving rise to the isopentenyl pyrophosphate isoprene unit (IPP) (FIGURE 5)[9].
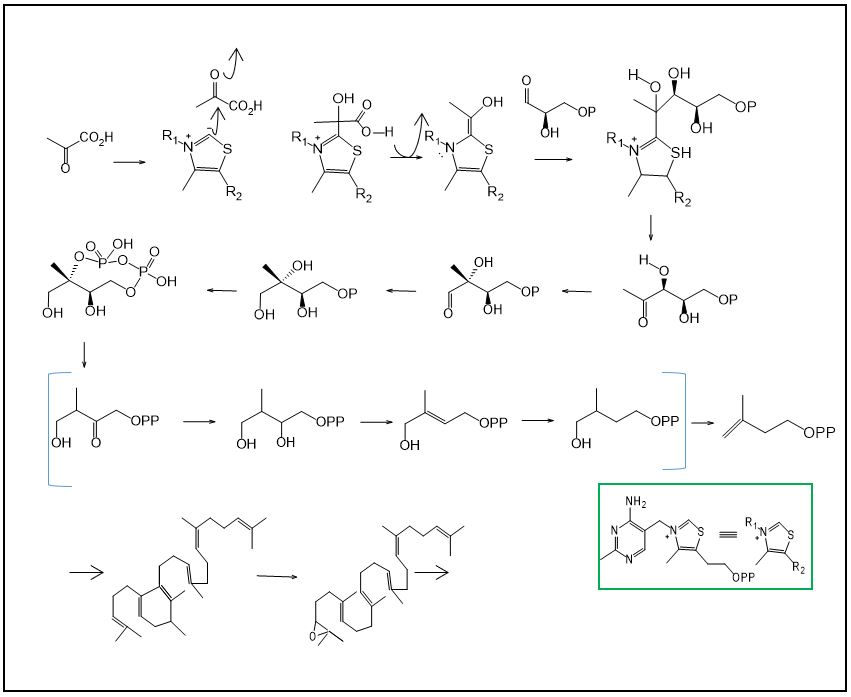
As in the mevalonate pathway, an isomerase removes a proton in the C-2 of the IPP, resulting in dimethylalyl pyrophosphate (DMAPP). Through condensation between these two units, gernanyl pyrophosphate (GPP) is generated. The reaction of this compound with another unit of isopentenyl pyrophosphate (IPP) gives rise to farnesyl pyrophosphate (FPP). The tail-tail condensation of two FPP units gives rise to a hydrocarbon formed by 30 carbon atoms called squalene, a precursor to triterpene compounds[5]. The cyclization of squalene occurs from the intermediate 2,3-squalene epoxide and saponins are formed through successive reactions, hydrides, methyls and carbocations[5].
Biological properties and activity-structure relationship
Structure-activity relationships (SAR) explore the relationship between a molecule's biological activity and the three dimensional structure of the molecule. Understanding structure–activity relationships for a given set of molecules it is important because allows one to explore chemical space and develop a chemical series optimizing multiple physicochemical and biological properties simultaneously[11].
Triterpenoidal saponins are bioactive compounds and over the years has been reported many studies about their biological properties, where is widely described the anti-viral, adjuvant, hemolytic, cytotoxic and anti-angiogenic activities that are relationship with the presence of characteristics chemical moiety. Recent works have suggested some triterpenoid saponins like candidates for treatment of patients with COVID-19. In this review are described the biological properties as well as structure activity relationships (SAR) of the triterpenoidal saponins
Anti-SARS-CoV-2
The pandemic of novel Corona Virus Disease 2019 (COVID-19) caused by SARS-CoV-2 virus has resulted in over 114,428,211 million confirmed cases of infections and over 2,543,755 million deaths worldwide[12], which accentuate the need for drugs discovery and deployment efforts to get available treatments. Several COVID-19 vaccines have now been authorised or approved for human use, with many more in the late stages of clinical development. Yet having licensed vaccines isn't enough to achieve global control of COVID-19: they also need to be produced at scale, priced affordably, allocated globally so that they're available where needed and widely deployed in local communities[13].
Given the alarming situation in which the world finds itself, substances have been emergencyly researched to end the new coronavirus. In addition to the use of vaccines, there are few compounds such as lopinavir, ritonavir, chloroquine, hydroxychloroquine and remdesivir have advanced to clinical trials, but they exhibited the limited curative effect and toxic side effects for the treatment of COVID-19 patients[14,15]. Aiming at the discovery of an effective drug against the SARS-CoV-2 virus, as well as a new alternative in the treatment against COVID-19, research is found in the literature with the aimed to develop specific anti-SARS-CoV-2 agents offering efficacy and safety, which can be used alone or in combination with vaccines in future infections[16].
In literature was reported that a focused screen of oleanolic acid saponins identified 3-O-β -chacotriosyl oleanolic acid benzyl ester (FIGURE 6) as a novel small molecule inhibitor of SARS-CoV-2 virus entry, via binding to SARS-CoV-2 glycoprotein (S)[14].
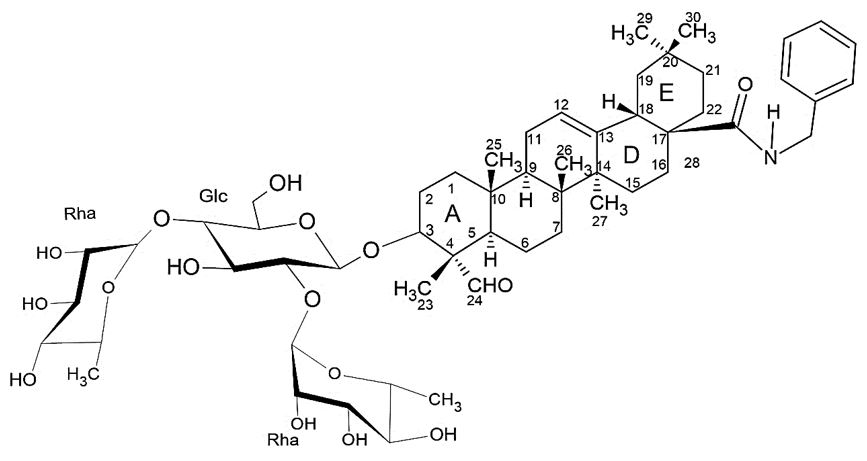
It is known that the superficial structural spicule glycoprotein (S) of the SARS-CoV-2 virus plays a vital role in mediating the entry of host cell viruses that is the first step in the infection process[14,15]. Structurally, SARS - CoV-2 S glycoprotein exists as a homotrimer with several glycosylation consists of two subunits, a N-terminal S1 subunit and a C-terminal S2 subunit. Afterwards of the Subunit SARS-CoV-2 S1 recognize the ACE2 cellular catcher through the receiver connection domain (RBD), Subunit S2 will change its conformation to adjust S mediated viral/cellular membrane merger[14,16,17]. Blocking Glycoprotein S may hinder SARS-CoV-2 entry into cell and downstream replication processes that provides important clues to overcoming SARS-CoV-2. The SARS-CoV-2 S protein is of great interest to antiviral development due to its ability to connect with the human ACE2 receiver, generating membrane fusion events that take it possible the virus to enter host human beings cells.
Oleanolic acid saponins were found to show a variety of inhibitory activities against SARS-CoV-2, strongly related to their structures. A preliminary significant structure-activity relationships (SARs) could be drawn from these observations to support further chemical optimization. In this research was analyzed the effect of branched trisaccharide scaffold of oleanane-type triterpenoid saponin on them inhibition. It was found that removal or positional change of its α-Lrhamnosyl residues just like replacement of its α-L-rhamnosyl or β-D-glucopyranosyl residue with other monosaccharides, stemmed in a significantly declined inhibition and even a overall loss of potency. These results hinted that the chacotriosyl moiety was essential for inhibitor efficacy[14].
Despite the discovery of saponin as a potent inhibitor of SARS-CoV-2 virus, was observed moderate cytotoxicity against 293T-ACE2 cells. To cease toxic side effects, has been incorporated into chemical modifications in the structure triterpenoid saponin. Thus, it was attempted to adopt the amide linker to replace the ester link in a position C-17, to improve the selectivity index.
Concurrently to bioisosteric substitution to retire cytotoxicity, modifications have been made to affinity with SARS-CoV-2-S. Thus, it was designed and synthesized a series of C-28 amide-replaced 3-O-b-chacotriosyl derivatives, anticipating that phenyl and aralkyl groups replaced in twenty-eight a position would have enough length and size, flexibility as well as hydrophobicity to effectively bind to the hydrophobic potential (FIGURE 7)[14].
The 12f saponin (FIGURE 7) stood out among all title saponins evaluated, displaying excellent anti-SARS-CoV-2 activity and low cytotoxicity to 293T-ACE2 cells, which could be chosen as the lead compound for SARS-CoV-2 entry inhibitors[14,15]. This saponin was capable of inhibiting SARS-CoV-2 infection in a dose-dependent manner as do the control group, confirming the effect anti-SARS-CoV-2.
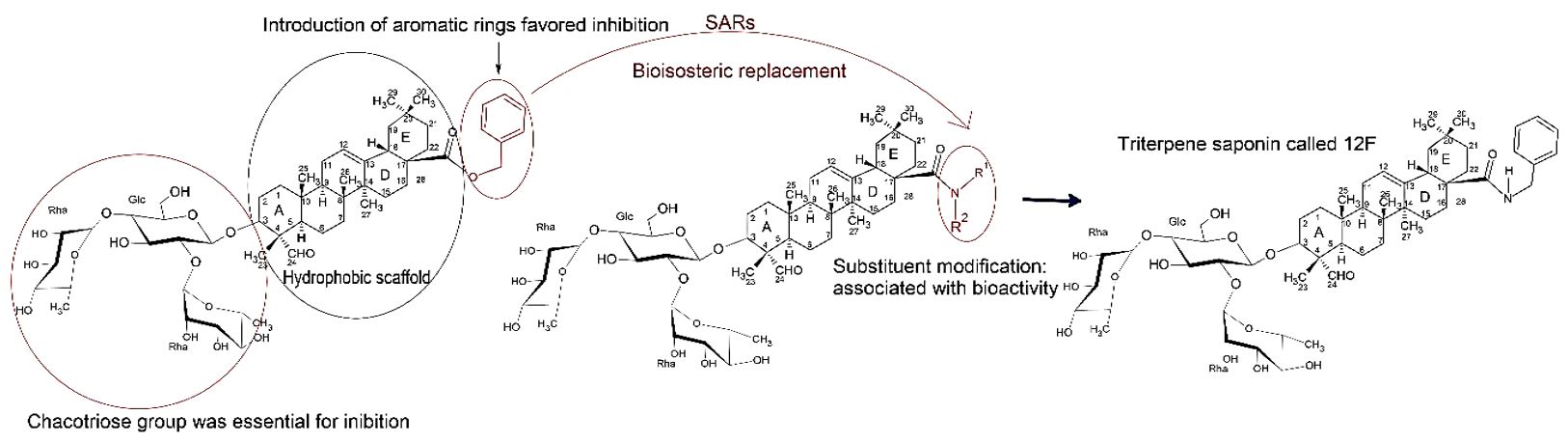
Collectively, the saponin 12f was an improved lead compound in relation to the hit 2, which was a good candidate as a potential antiviral treatment for SARS-CoV-2[14]. In summary, the authors related that triterpenoidal saponin 12f had favorable SI values when tested with infectious SARS-CoV-2. Intensive SARs indicated that the chacotriosyl moiety was indispensable for efficacy and introduction of a hydrophobic aromatic ring through a flexible amide linker at 28-position of oleanolic acid favored antiviral activity and selectivity rate. Utilizing the Co-IP assay, Surface Plasmon Resonance analysis and cell-cell fusion studies, it was confirmed that the lead 12f was capable of direct binding to SARS-CoV-2 S2 subunit[14].
Some hypotheses are found in the literature that others saponins can help in the treatment of symptoms caused by COVID-19. It is reported that triterpenoid saponins Saikosaponin A, Saikosaponin B, and Saikosaponin D from Bupleurum falcatum L. can be candidate to treatment for COVID-19 owing to their anti-inflammatory, immunomodulatory, and antiviral activities. The authors recommend future welldesigned randomized controlled trials to evaluate the safety and efficacy of Saikosaponins in patients with COVID-19[18].
Glycyrrhizic acid, is a triterpene saponin non-hemolytic, potent immuno-active anti-inflammatory agent, It is used to treat liver diseases and specific cutaneous inflammation. The anti-coronavirus potential of the glycyrrhizic acid (GLR) was investigated and showed activities against SARS-associated Human and animal corona viruses. The authors conclude that glycyrrhizic acid should be further considered and rapidly evaluated for the treatment of patients with COVID-19[19].
Adjuvant activity
Vaccine adjuvants are compounds that enhance/prolong the immune response to a co-administered antigen. Saponins have been widely used as adjuvants for many years in several vaccines - especially for intracellular pathogens - including the malaria and shingles vaccines[20]. The search for natural adjuvants is increasing aiming at promising substances and low toxicity. The first report of triterpenoid saponins with adjuvant activities were the constituents present in the Quillaja saponaria Molina tree, a species native to Chile, known to cause a substantial increase in the immune response where its extracts are used in veterinary vaccines such as Leshimaniose and feline Leukemia[21,22].
As an example of complex triterpenoid saponin with adjuvant activity one has the QS-21, which is a natural triterpenoid saponin extracted from the barks of Q. saponaria Molinaand is one of the most potent adjuvants known which is currently used in exploratory, and a few licensed, vaccines. The QS-21 is a mixture of two isomeric molecules, QS-21 Apiose (QS-21 Api), and QS-21 Xylose (QS-21-Xyl) (2:1), each having four domains: the triterpene quillaic acid, a branched trisaccharide linked at C-3 through an O-heterosidic bound, a linear tetrasaccharide linked at C-28 through an ester bound, and a glycosylated pseudodimeric acyl chain attached through a labile ester linkage at C-4 of the fucose unit of the tetrasaccharide (FIGURE 8)[23].
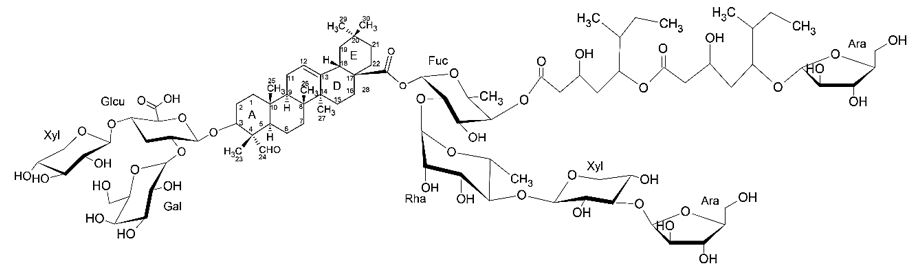
In many adjuvants the identification of chemical groups that interact with specific cell receptors for specific innate or natural immunity allows the establishment of the structure-function relationship that are useful in the design of new adjuvants[23]. It has been observed in the scientific literature that complex triterpenoid saponins produce a good immune response. The aldehyde chemical function present in C-4 of Quillaja saponins in general, are responsible for the interaction of auxiliary T lymphocytes and the induction of th1 cellular immune response[23].
It is reported a study where was investigated the humoral immune response in the purified fractions QS-21 (F1), Desacylaponins (F2), Sucrose (F3) and Glucose (F4) (FIGURE 9) obtained from a commercial extract of a mixture of Riedel De Haen® saponins. In this work, mice were vaccinated with 3 weekly doses of Leshimaniasis antigen (Leishmania donavani) with each of the purified fractions. After immunization the animals were challenged with an intravenous injection of Leshimania infantum chagasi, in which an increase in all immunoglobulin isotypes for QS-21 (F1) was observed. A similar increase in desacylsaponins (F2) can be observed only for the IgG1 isotype, which shows that the monoterpenic part in C-28 promoted a higher humoral immune response with different immunoglobulin isotypes. It has also been observed that while the aldehyde group present in Quillaja saponins is crucial to stimulate a Th1 cellular immune response, the hydrophobic group bound in C-28 is responsible for stimulating the production of cytotoxic T-cell response against exogenous proteins and is related to undesirable toxic effect[24].
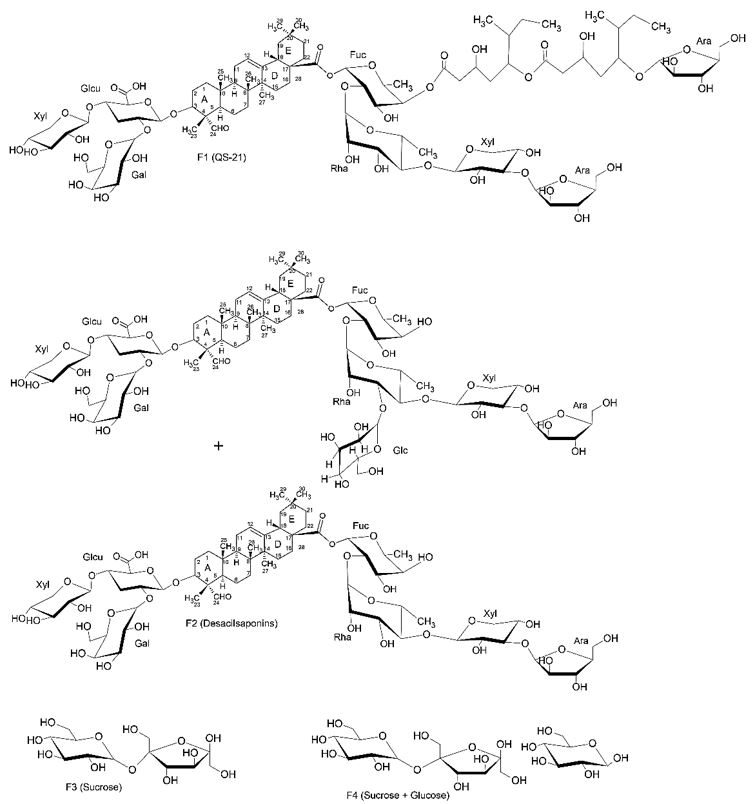
The first detailed immunological study of saponin fractions isolated by High Performance Liquid Chromatography was using bovine serum albumin as antigen and showed that the main peaks QS-7, QS-17, QS-18 and QS-21 have adjuvant activity. Little is known about the activity and structure of hydrophilic saponin QS-7 (FIGURE 10). Like QS-21, QS-7 saponin is shown to be bidesmosidic, being glycosylated in C-3 and C-28, has a higher degree of glycoylation, being more polar and has only one acile unit considerably smaller than the other saponins of Q. saponaria tree[25].
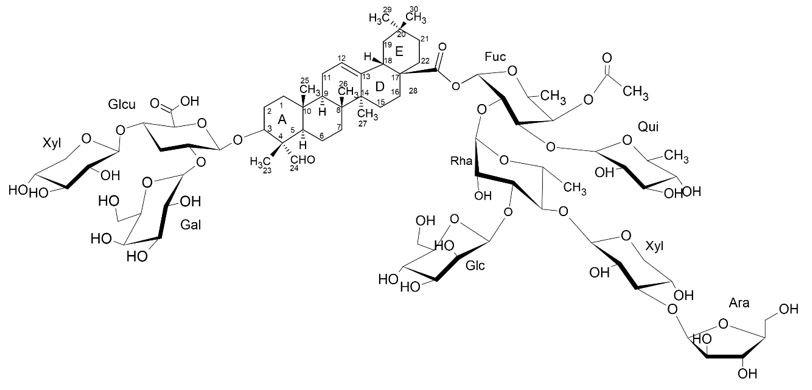
It was observed in the scientific literature that unlike QS-21, saponin QS-7 exhibited negligible toxicity in guinea pigs, however, it doesn't have many clinical advances due to difficulties in its purification and the uncertainty of its structural constitution[26].
The toxic properties of Quillaja saponins limit their use as adjuvants in the development of vaccines, in which, although these saponins have physical properties and adjuvants similar to their toxicity is very varied. It has been observed in the literature that saponins QS-17 and QS-18 are highly toxic compounds and from the comparison of these saponins with QS-21 it is revealed that the only significant structural difference in both saponins is the presence of a glucose linked to rhamnose present in fucose residue (FIGURE 11)[27,28].
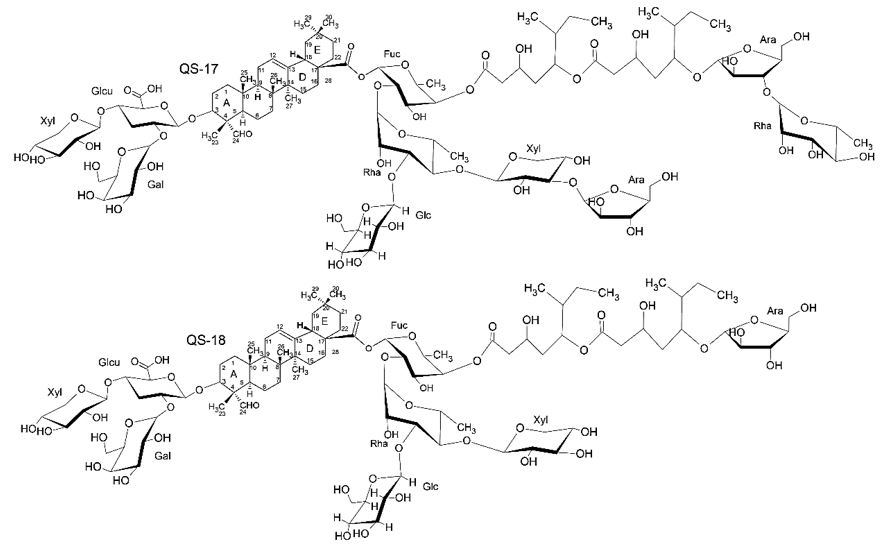
It can be observed that both saponins are glycosylated in C-3 and C-28, being bidesmosidic, have an aldehyde group in C-4, have the same glycosidic parts in C-3 and two monoterpenic parts connected linearly located between the carbohydrates arabinosis and fucose[27].
The AS01 is unique amongst other adjuvants as it contains two discrete immunostimulatory molecules known to have adjuvant properties on their own, 3-O-desacyl-4′-monophosphoryl lipid A (MPL) and the saponin QS-21. MPL and QS-21 are formulated together in liposomes in the presence of cholesterol in order to abrogate the hemolytic activity of QS-21. AS01 has now been evaluated in several candidate vaccines and shown to consistently increase both antibody and T-cell responses, regardless of the antigen used, and age or specific immune conditions involved. Importantly, this enhanced immunogenicity translated into clinical efficacy which allowed the development of the vaccine against Plasmodium falciparum malaria, and the vaccine against herpes zoster (vaccine registered)[28].
Saponins isolated from Quillaja brasiliensis Mart., a native tree from southern Brazil and Uruguay, have been thoroughly studied as a promising alternative to the development of new vaccine adjuvants. The saponin fractions, named QB-80, have confirmed its potential as immune adjuvants when added to a number of distinct viral antigens in murine models. Additionally, these fractions show substantial chemical similarity to Quil A® triterpenoid saponin, with the advantage of inducing fewer toxic effects than Quil A. QB-80, an immunoadjuvant obtained from leaf extract of Quillaja brasiliensis, has at least 29 triterpene saponins which were provisionally identified by the DI-ESI-ToF[28].
These saponins are capable of self-assembly with cholesterol and di-palmitoylphosphatidyl hill to form ISCOM-like nanoparticles with approximately 43 nm, called IMXQB-80. QB-80 SBAs significantly increase immune responses to an experimental ZIKV vaccine. The IMXQB-80 nanoadjuvant formulation, which contains four times less saponins, however proved that be as effective as an immune enhancer as QB-80, with the advantage to mitigate the toxic side effects usually associated with SBAs[28].
The activity of the immunological adjuvant was revealed by a significant increase serum IgG levels and anti-ZIKA virus subtypes (IgG1, IgG2b and IgG2c), as well as neutralizing antibodies, when compared with a vaccine without adjuvant. Overall, these findings reveal that QB-80 or IMXQB-80 are strong immunoadjuvants and have shown be more potent immune stimulators than alum, a widely used vaccine adjuvant[28].
Hemolytic activity
Saponins have the ability to break through erythrocytes, releasing hemoglobins that consist of a cell compartment called red blood cells. Hemolytic properties are directly related to the interaction between the nonpolar part of saponin, such as its aglycone nucleus (for steroidal and triterpenoid saponins, as well as the monoterpenic parts and its hydrophobic nucleus for complex triterpenoid saponins) with sterols present in the lipid bilayer of the cell membrane[29].
Saponins form complexes with the steroids of the lipid bilayer of the erythrocyte membrane in which the aglycone (hydrophobic) part of the saponins interacts with the membrane cholesterol inserting into the membrane and the glycosidic part (hydrophilic) interacts with the glycoproteins present on the membrane surface[29].
From the mechanism of functionality of the cell membrane it is possible to obtain a better understanding of hemolytic activity whose steric interference of the glycoside sections causes the curvature of the membrane leading to (A) consisting of the formation of pores in the membrane or the formation of (B) consisting of hemitubular protubeances resulting in the extraction of cholesterol through vesiculation. Or even leading to (C), in which there is another current of researchers who argue that breaking a cell membrane only by interacting with cholesterol would be a very drastic effect, with little cholesterol in a membrane to break it, and it requires additional interactions with the fatty acids present in the membrane phospholipid chain. Cell membrane rupture is related to increased saponin concentration, responsible for increased membrane permeability (FIGURE 12)[30,31].
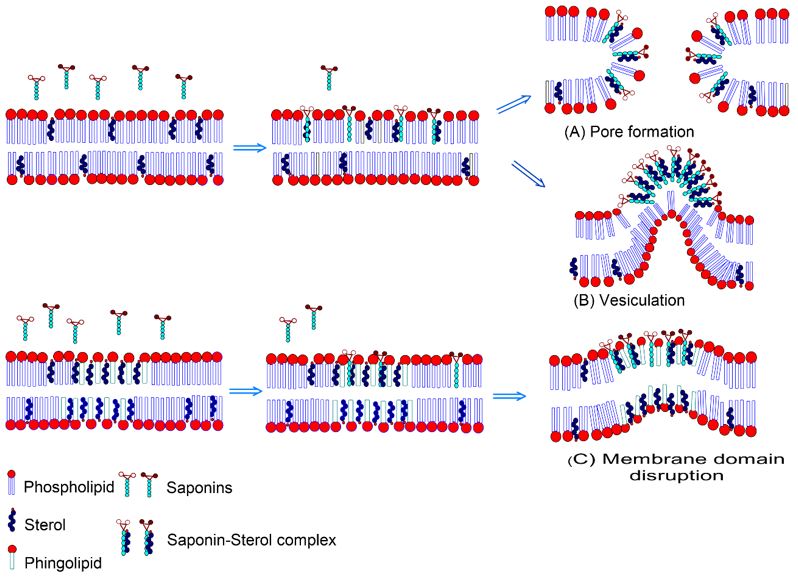
It is reported in the literature that complex triterpenoid saponins are generally more hemolytic than triterpenoid saponins due to a greater amount of aglycone and monoterpenic hydrophobic parts that interact with membrane cholesterol. QS-21 is an example of complex triterpenoid saponin with hemolytic activity (FIGURE 9).
Anti-angiogence activity
The Angiogenesis, which involves multiple cells and soluble factors for the formation of blood vessels from the preexisting ones, plays a pivotal role in the process of tumor growth and metastasis blocking angiogenesis is a validated effective therapeutic approach against cancer and divers antiangiogenic agents have been successfully translated into cancer clinic. Beyond the approved monoclonal antibody and small-molecule tyrosine kinase inhibitors (TKIs), natural products from medical herb are attracting a growing amount of researchers to excavate their antiangiogenic activity[29,30].
It is described in the literature a study that revealed the antiangiogenic potency of Raddeanin A (RA) oleanane-type triterpenoid saponin (FIGURE 13) extracted from the roots of Anemone raddeana Regel, a traditional Chinese medicinal herb[31].
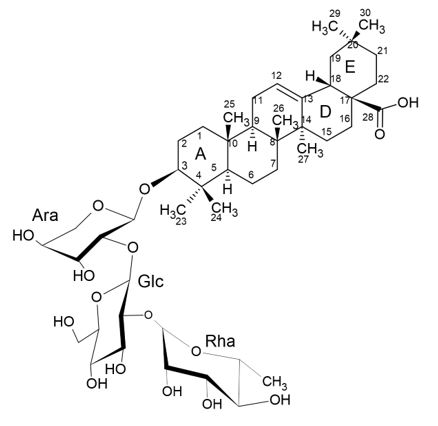
The antiagiogenic activity of RA triterpenoid saponin has been shown using human umbilical vein endothelial cell (HUVEC), chick chorioallantoic membrane (CAM) model, and transgenic zebrafish angiogenesis model. The tumor antiangiogenic efficacy of RA was evaluated in the subcutaneous HCT-15 xenograft mice model. Furthermore, the antiangiogenic molecular mechanism of RA was explored by western blot and molecular docking assay[29].
Antiangiogenic intervention mediated by natural products is a promising research area that provides an effective anticancer strategy. Many natural compounds have been proved to possess antiangiogenic activity in vitro and in vivo. In this study was found that RA triterpenoid saponin possessed remarkable antiangiogenic effect both in vitro and in vivo. RA triterpenoid saponin interrupted a series of orchestrated processes of angiogenesis, including EC proliferation, motility, migration and capillary-like tube formation in a dose-dependent manner, and inhibited in vivo chick CAM angiogenesis[29,30].
Transgenic zebrafish was also adopted to further evaluate the antiangiogenic activity of RA triterpenoid saponin. The quantification of the number of complete intersegmental vessels (ISVs) is one of the reliable parameter to evaluate in vivo angiogenesis. RA could inhibit the ISVs formation of zebrafish in a non-lethal dose. The authors described that RA triterpenoid saponin effectively disrupted the completion of ISVs and only occasional sprouts of dorsal aorta were observed. Both PTK787 (positive control) and RA significantly blocked blood vessel formation, which may be responsible for the observed pericardial edema. It's also noted that the morphology of zebrafishes treated with RA deformed and curved to some extent[29,30].
Prompted by the robust antiangiogenic activity of RA triterpenoid saponin, that a other research described that was applied RA inhibited HCT-15 xenograft tumor growth through antiangiogenic effect to investigate the effect of RA on tumor growth of human colon rectal cancer, nude mice bearing subcutaneous HCT-15 xenograft were treated with 5mg/kg RA Every other day for total 11i.p. injections. It showed that RA significantly inhibited HCT-15 tumor growth and decreased the tumor size. The tumor volume in RA-treated group was 765.3±156.6 mm3, smaller than control group (1919.0±670.6 mm3). The average tumor weight of control group was 2.2±0.5g, whereas that of RA treated group was only 1.2±0.3g. The mice weight loss after RA treatment wasn't significant, suggesting that RA didn't cause obvious toxicity to the mice at curative dose. Immunohistochemical and pathological examinations showed that RA treatment significantly decreased intratumoral MVD, elevated TUNEL-positive cells, and led to increased tumor necrotic area compared with the control group. These results showed that the antitumor effect of RA was related to its antiangiogenic activity. The data obtained suggest that the antiangiogenic activity presented by saponin is due to the nature of its oleanane-type sapogenin[30].
Cytotoxic activity
Oleanane-type triterpenoid saponins named Erythrosides B and Erythrosides C, were isolated from the seeds of Erythrophleum fordii Oliver (Leguminosae). Erythrosides B-C saponins fatured several oligosaccharide chain containing 5–6 hexoses at C-3 and a monoterpenic acid at the C-21 position[31,32]. In addition to the monoterpenic part, Erythrosides B-C complex triterpenoid saponins contained a rare alpha xylose in their sugar chains (FIGURE 14)[33].
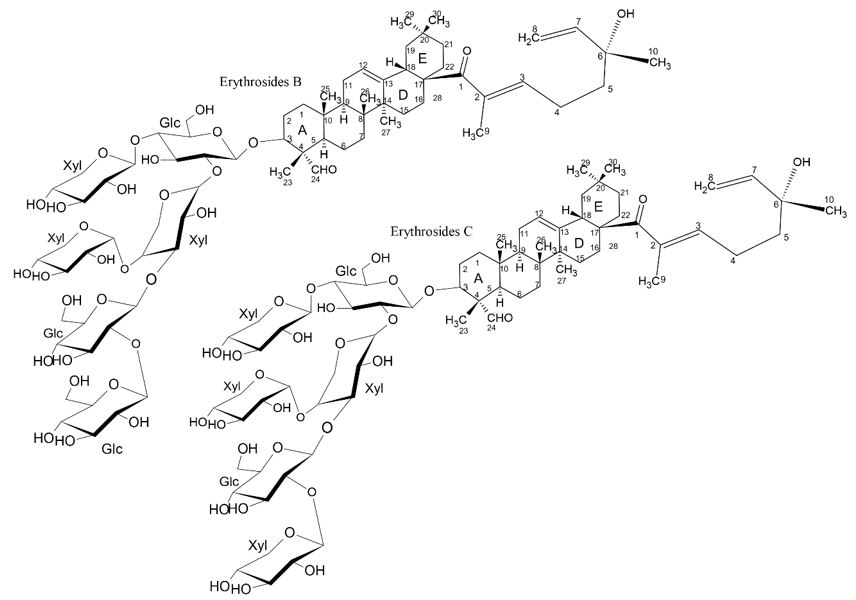
In this study, the cytotoxicity of isolated compounds was investigated using the MTT assay in the HCT-116 human colon cancer cell line, the human glioblastoma cell line U87-MG, the human liver cancer cell line Hep-G2, the line of human gastric cancer cells BGC-823 and the human lung PC-9 cancer cell line (TABLE 1). The results showed that Erythrosides B-C complex triterpenoid saponins, significantly suppressed the proliferation of PC-9 cancer cells with IC50 values of 13.14, 16.67 and 17.59 μM, respectively, while the positive Taxol control exhibited an IC50 of 0.60 nM. The saponins showed a selective and moderate cytotoxic activity against the line of human pulmonary PC-9 cancer cells, whose bioactivity may be related to the presence of the monoterpenic portion bound to aglycone in C-21, as well as due to the presence of the rare alpha xylose carbohydrate (TABLE 1)[31].
Compound | Cell lines (IC50 µmol.L-1) | ||||
HCT-116 | U87-MG | HepG-2 | BGC-823 | PC-9 | |
Erythroside B | 25.46 | > 50.0 | 28.61 | 46.24 | 16.67 |
Erythroside C | 38.65 | 29.04 | 35.46 | > 50.0 | 13.14 |
Taxola (nmol.L-1) | 0.000389 | 0.006757 | 0.007143 | 0.001098 | 0.000603 |
aTaxol was used as a positive control to cytotoxic assay Source: Table adapted from[31]. |
In another study, a comparison was made between how to cytotoxicities (ED50) measures against a variety of tumor cell lines of extracts of Gleditsia aquatica Marshall and two complex saponins (FIGURE 15) isolated from this same species[32].
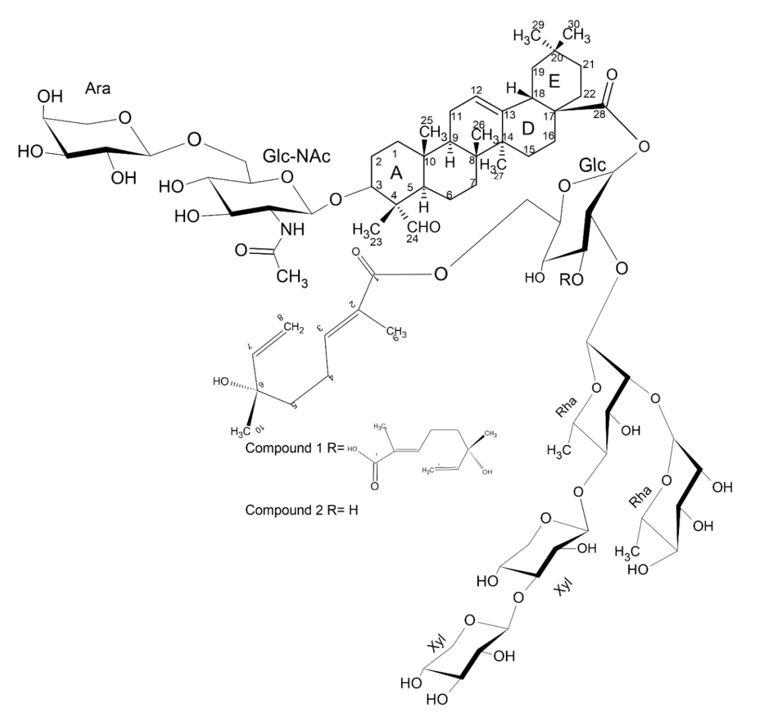
In the table below (TABLE 2) it can be observed that complex saponins 1 and 2 isolates of G. aquatica show more potent cytotoxicities in cell lines related to adenocarcinoma (MDAH-2774, NIH: OVCAR-3), mesodermal (SK-UT-1), melanoma (G-361) and colorectal adenocarcinoma (LS-174)[32].
Tumor cell lines ED50 (µg/mL) of extracts and pure compounds 1 and 2 of G. aquatica EtOH ETOAc n-BuOH 1 2 |
|||||
Liver NCI-H1755 | 77.39 | > 100 | 80.65 | 70.87 | 83.66 |
Tumor 1-SK-HEP | > 100 | 65.93 | > 100 | 54.93 | 73.18 |
Kidney carcinoma A-498 | > 100 | > 100 | > 100 | > 100 | > 100 |
Kidney SW 156 | 92.45 | 65.91 | > 100 | 48.94 | 64.23 |
Ovarian MDAH 2774 | 17.47 | 13.03 | 30.75 | 4.86 | 5.45 |
NH:OVCAR | 23.81 | 26.19 | 39.15 | 3.11 | 14.66 |
Mesodermal uterus SK-UT-1 | 8.93 | 12.17 | 27.68 | 6.23 | 3.68 |
Malignant Melanoma G-361 | 13.34 | 16.43 | 25.72 | 10.15 | 9.95 |
RPMI-7951 | 35.65 | 12.76 | 28.49 | 11.40 | 12.15 |
HS 908-SK | 48.14 | 55.15 | 45.85 | 58.82 | 65.25 |
Prostate Carcinoma DU 145 | 42.12 | 28.14 | 25.00 | 36.81 | 32.03 |
Colorectal HCT8 | 67.74 | 59.26 | 45.75 | 42.70 | 40.38 |
LS 174T | 40.78 | 32.18 | 29.80 | 4.18 | 3.66 |
Colon 320 DM | 82.05 | 75.15 | 90.35 | 48.60 | 52.85 |
Source: Table adapted from[32]. |
In addition, there are reports in the literature that acyl saponins isolated from the fruits of Gleditsia sinensis Lam. and Gleditsia capsica Desf. show variable cytocities when tested against human tumor cell lines. In the structure-activity relationship, the monoterpenic parts of complex saponins were crucial for cytotoxic activity and the presence of a hydroxyl substitut in the hydrophobic part in C-16 is responsible for increasing activity. Some authors argue that the increase in the number of monoterpenic units attached to the terminal of the α-L-rhamnopyranosil part of the chain of oligosaccharides in C-28 increases activity while introducing a sugar sofarinto the monoterpenic structure seems to have no effect on the activity[33].
Commercial applicability of triterpenoidal saponins
Due to their potent biological activities, triterpenoidal saponins have a wide commercial application. However, for the saponin be used in the medicine, and there should be clinical trials in relation to its activity and toxicity.
It is reported that saponins can be application in foods and cosmetics, but it is necessary that sufficient amounts of plant resources are available, and that the content of saponins must be high. Furthermore, the vegetal material must have a wide history of human use as foodstuffs or ingredients of cosmetics and their safety should be officially guaranteed[34].
Despite the vast presence of triterpenoidal saponins in plants, there are few saponins used as isolated compounds, standing out glycyrrhizin from Glycyrrhiza glabra L.[34] and QS-21 from Q. saponaria Molina[23] , which are used to treat problems in the liver and in vaccines respectively.
The TABLE 3 shows some isolated saponins, mixture of saponins or extract contend triterpenoidal saponins that are present in the composition of commercial products.
Saponins | Specie plant | Commercial applicability | Reference |
QS-21 | Q. saponaria Molina | Veterinary vaccine | [23] |
Quillaja saponins | Q. saponaria Molina | Surfactant, food ingredients, shampoos, detergents, toothpastes, pharmaceutical application as suspension stabilizer | [34,35] |
Glicirrizin | G. glabra | Herbal medicine on the liver Sweetener and flavor enhancer in foods and cigarettes | [36,34,37] |
Sapindus saponins | Sapindus mukorossi Gaerth | Ingredient in cosmetics | [34] |
Jua bark saponins | Z. joazeiro Mart | Detergent, dentifrice and phytotherapic | [38] |
Asiaticoside | Centella asiatica | Herbal medicine in wound healing | [39] |
Ginsenosides | Panax ginseng Meyer | Herbal medicine to treat inflammation | [40] |
Final considerations
Complex triterpenoidal and triterpenoidal saponins stand out for presenting various biological activities that are related to interactions of specific chemical groups of these substances with other molecules, such as cell receptors. In many studies, the influence of the monoterpenic part on a strong biological activity and at the same time on undesirable toxicity has been observed. This is due to the larger hydrophobic part that interacts with the cholesterol sections of the cellular lipid bilayer. For Quillaja saponins, the adjuvant will mediate the recognition of the antigen by lymphocyte, neutrophil or antigen-presenting cells, through the aldehyde group present in the C-4 of its sapogenin, with a more pronounced aduvanite activity for QS-1 due to the addition of monoterpenic and glucuronic acid. As in many saponins, carbohydrate stakes can be fundamental to sustain a given activity, and there may be changes in key groups to inhibit toxicity and potentiate certain biological activity. The structure-activity will influence the commercial application of the product, and the substance may be a strong candidate for the vaccine, medicine, and there should be clinical trials in relation to its activity and toxicity.
Conclusion
In this review article it was possible to gather the works that the authors judged most important within this proposal to emphasize the importance of each chemical group present in a molecule. Chemical groups can act as active sites, as well as functional portions, directly interfering in biological activity and consequently in its application as a medicine. For a product to become a drug it is necessary a series of researches, in vitro and in vivo tests, clinical trials, in addition to research on toxicity. Saponins have been shown to have several active sites that will interact with other molecules influenced by biological activity. From this it was possible to observe that triterpene sapogenin, as well as the chemical groups present in these, the nature/composition of carbohydrates and the presence or absence of monoterpence poreds were fundamental for the determination of biological activities and will consequently influence its commercialization as a final product, be it medication or vaccine.
Funding Source
There was no funding.
Conflicts of interest
The authors declare that they have no conflict of interest.
Contribution
Study design: MFSJC
Data curation: MFSJC; GMP
Data collect: MFSJC; GMP
Data analysis: MFSJC; GMP
Original manuscript writing: MFSJC; GMP
Revision writing and editing: MFSJC; GMP.
References
1. Zhou W, Yang J, Lou L, Zhu L. Solubilization properties of polycyclic aromatic hydrocarbons by saponin, a plant-derived biosurfactant. Environ Pollut. 2011; 159(5): 1198-1204. ISSN 0269-7491. [https://doi.org/10.1016/j.envpol.2011.02.001] [https://pubmed.ncbi.nlm.nih.gov/21353355/].
2. Vincken J-P, Heng L, Groot A, Gruppen H. Saponins, classification and occurrence in the plant kingdom. Phytochemistry. 2007; 68(3): 275-297. ISSN 0031-9422. [https://doi.org/10.1016/j.phytochem.2006.10.008] [https://pubmed.ncbi.nlm.nih.gov/17141815/].
3. Ashraf MF, Abd Aziz M., Stanslas J, Ismail I, Abdul Kadir M, Assessment of antioxidant and cytotoxicity activities of saponin and crude extracts of Chlorophytum borivilianum. Sci World J. 2013; 2013: 1-7. ISSN 1597-6343. [https://doi.org/10.1155/2013/216894].
4. Wina E, Muetzel S, Becker K. The impact of saponins or saponin-containing plant materials on ruminant production: a review. J Agric Food Chem. 2005; 53(21): 8093-8105. ISSN 1520-5118. [https://doi.org/10.1021/jf048053d].
5. Simões CMO, Schenkel EP, Mello JCP, Mentz LA, Petrovick PR. Farmacognosia: do produto natural ao medicamento. 1ª Edição. Porto Alegre: Artmed; 2017. ISBN 978-85-8271-359-4.
6. Cruz MFSJ, Pereira GM, Ribeiro MG, Silva AM, Tinoco LW, Silva BP et al. Ingasaponin, a complex triterpenoid saponin with immunological adjuvant activity from Inga laurina. Carbohydr Res. 2016; 420: 23-31. ISSN 0008-6215. [https://doi.org/10.1016/j.carres.2015.11.008] [https://pubmed.ncbi.nlm.nih.gov/26717546/].
7. Pawan MD, Shingade SH, Chandu MS. Comparative study of saponin for surfactant properties and potential application in personal care products. Material today: proceedings. 2021; 45 (3): 5010-5013. ISSN 2214-7853. [https://doi.org/10.1016/j.matpr.2021.01.448].
8. Wei MP, Qiu JD, Li L, Xie YF, Yu H, Guo YH et al. Saponin fraction from Sapindus mukorossi Gaertn as a novel cosmetic additive: extraction, biological evaluation, analysis of anti-acne mechanism and toxicity prediction. J Ethnopharmacol. 2020; 268: 113552. ISSN 0378-8741. [https://doi.org/10.1016/j.jep.2020.113552].
9. Lobo AM, Lourenço AM. Biossíntese de Produtos Naturais. 1ª Edição. Lisboa Portugal: Editora IST Pres; 2007. ISBN: 9789728469504.
10. Dewick PM. Medicinal Natural Products: A Biosynthetic Approach. 3rd Edition. West Sussex: Wiley; 2002. ISBN 0-471-49640-5.
11. Guha R. On Exploring Structure–Activity Relationships. In: Kortagere S. (eds) In Silico Models for Drug Discovery. Methods Mol Biol Humana. Press, Totowa, NJ. 2013; 993: 81-94. ISBN 978-1-62703-342-8. [https://doi.org/10.1007/978-1-62703-342-8_6].
12. World Health Organization (WHO). Guidelines for Coronavirus disease (COVID-19): weekly epidemiological update and Weekly operational update (2021). Disponível em: [https://covid19.who.int/]. [Acesso em: 1 out. 2021].
13. Li H, Cheng C, Li S, Wu Y, Liu Z, Liu M et al. Discovery and structural optimization of 3-O-b-chacotriosyl oleanane-type triterpenoids as potent entry inhibitors of SARS-CoV-2 virus infections. Eur J Med Chem. 2021; 215: 1-14. ISSN 0009-4374. [https://doi.org/10.1016/j.ejmech.2021.113242].
14. Krammer F. SARS-CoV-2 vaccines in development. Nature. 2020; 586: 516-27. ISSN 0028-0836.[https://doi.org/10.1038/s41586-020-2798-3] [https://www.nature.com/articles/s41586-020-2798-3].
15. Awadasseid A, Wu YL, Tanaka Y, Zhang W. Current advances in the development of SARS-CoV-2 vaccines. Int J Biol Sci. 2021; 17(1): 8-19. ISSN 1449-2288. [https://dx.doi.org/10.7150%2Fijbs.52569] [https://www.ijbs.com/v17p0008.htm].
16. Wouters OJ, Shadlen KC, Pollard AJ, Larson HJ, Teerawattananon Y, Jit. M. Challenges in ensuring global access to COVID-19 vaccines: production, affordability, allocation, and deployment. Lancet. 2021; 397: 10278, P1023-1034. ISSN 1474-547X. [https://doi.org/10.1016/s0140-6736(21)00306-8].
17. Lacaille-Dubois M.-A. Updated insights into the mechanism of action and clinical profile of the immunoadjuvant QS-21: a review. Phytomedicine. 2019; 60: 152905. ISSN 0944-7113.[https://doi.org/10.1016/j.phymed.2019.152905] [https://www.ncbi.nlm.nih.gov/pmc/articles/PMC7127804/].
18. Bahbah EI, Negida A, Nabet MS. Purposing Saikosaponins for the treatment of COVID-19. Med Hypotheses. 2020; 140: 109782. ISSN 0306-9877. [https://doi.org/10.1016/j.mehy.2020.109782].
19. Bailly C, Vergote G. Glycyrrhizin: An alternative drug for the treatment of COVID-19 infectionand the associated respiratory syndrome. Pharmacol Ther. 2020; 214: 107618. ISSN 1532-6535. [https://doi.org/10.1016/j.pharmthera.2020.107618].
20. Cibulski S, Teixeira FT, Varela APM et al. IMXQB-80: A Quillaja brasiliensis saponin-based nanoadjuvant enhances Zika virus specific immune responses in mice. Vaccine. 2021; 39(3): 571-579. ISSN 2590-1362. [https://doi.org/10.1016/j.vaccine.2020.12.004] [https://pubmed.ncbi.nlm.nih.gov/33339669/].
21. Sun HX, Xie Y, Ye YP. Advances in saponin-based adjuvants. Vaccine. 2009; 27(12): 1787-796. ISSN 2590-1362. [https://doi.org/10.1016/j.vaccine.2009.01.091].
22. Marciani DJ, Vacine adjuvants: role and mechanisms of action in vaccine immunogenicity. Drug Discov. today, DDT, 2003; 20: 934-943. ISSN 1359-6446. [https://doi.org/10.1016/s1359-6446(03)02864-2].
23. Liu G, Anderson C, Scaltreto H, Barbon J, Kensil CR. QS-21 structure/function studies: effect of acylation on adjuvante activity. Vaccine, 2002; 20(21-22): 2808-810. ISSN 2590-1362.[https://doi.org/10.1016/s0264-410x(02)00209-8] [https://pubmed.ncbi.nlm.nih.gov/12034108/].
24. Oliveira-Freitas E, Casas CP, Borja-Cabrera GP, Santos FN, Nico D, Souza LOP et al. Acylated and deacylated saponins of Quillaja saponária mixture as adjuvants for the FML- Vaccine Against visceral Leishmaniasis. Vaccine. 2006; 24: 3909-3920. ISSN 0264-410X. [https://doi.org/10.1016/j.vaccine.2006.02.034].
25. Deng K, Adams MM, David GY. Synthesis and structure verification of the vaccine adjuvant QS-7-Api. Synthetic access to homogeneous Quillaja saponaria immunostimulants. J Am Chem Soc. 2008; 130(18): 5860-586. ISSN 1520-5126. [https://doi.org/10.1021/ja801008m].
26. Kensil CR. Immunomodulatory adjuvants from Quillaja soponins. Immunopotentiators in Modern Vaccines, 2006; 7: 109-122. ISBN 978-0-12-088403-2 [https://doi.org/10.1016/B978-012088403-2/50008-3].
27. Barr IG, Sjolander A, Cox JC. ISCOMs and other saponin based adjuvants. Adv Drug Deliv Rev. 1998; 32(3): 247-271. ISSN 0169-409X [https://doi.org/10.1016/s0169-409x(98)00013-1].
28. Augustin JM., Kuzina V, Andersen SB, Bak S. Molecular activities, biosynthesis and evolution of triterpenoid saponins. Phytochemistry. 2011; 72(6): 435-45. ISSN 0031-9422 [https://doi.org/10.1016/j.phytochem.2011.01.015].
29. Guana Y-Y, Liua H-J, Luana X, Xua J-R, Lua Q, Liua Y-R et al. Raddeanin A, a triterpenoid saponin isolated from Anemone raddeana, suppresses the angiogenesis and growth of human colo rectal tumor by inhibiting VEGFR2 signaling. Phytomedicine. 2015; 22(1): 103-110. ISSN 0944-7113 [https://doi.org/10.1016/j.phymed.2014.11.008].
30. Naz I, Ramchandani Sh, Khan MR, Yang MH, Ahn KS. Anticancer Potential of Raddeanin A, a Natural Triterpenoid Isolated from Anemone raddeana Regel. Molecules. 2020; 25(5): 1-20. ISSN 1420-3049 [https://doi.org/10.3390/molecules25051035].
31. Chena Z-P, Guo L-B, Heb J, Xu J-K, Lic Y-N, Huanga X-Y et al. Triterpene saponins from the seeds of Erythrophleum fordii and their cytotoxic activities. Phytochemistry. 2020; 177: 112428 ISSN 0031-9422. [https://doi.org/10.1016/j.phytochem.2020.112428] [https://pubmed.ncbi.nlm.nih.gov/32535346/].
32. Ragab EA, Hosny M, Kadry HM, Hassan A. Acylated triterpenoidal saponins and cytokinins from Gleditsia aquatica. J Pharmacogn Phytother. 2010; 2(3): 24-33. ISSN 2141-2502 [https://doi.org/10.5897/jpp.9000006].
33. Melek FR, Kassem IAM, Miyase T, Fayad W. Caspicaosides E-K, triterpenoid saponins and cytotoxic acylated saponins from fruits of Gleditsia caspica Desf. Phytochemistry. 2014; 100: 110-119. ISSN 0031-9422. [https://doi.org/10.1016/j.phytochem.2014.01.019]
34. Tamura Y, Miyakoshi M, Yamamoto M, Application of Saponin-Containing Plants in Foods and Cosmetics. Altern Med. 2012; 87-10. ISBN 978-953-51-7054-9 [https://doi.org/10.5772/53333].
35. Setten DC, Werken G. Molecular Structures of Saponins from Quillaja saponaria Molina. In: Waller GR, Yamasaki K. (eds) Saponins used in traditional and modern medicine: plenum press. 1996; 404: 185-193. ISBN: 978-1-4899-1367-8 [https://doi.org/10.1007/978-1-4899-1367-8_17] [https://pubmed.ncbi.nlm.nih.gov/8957295/].
36. Girish C, Pradhan SC. Herbal Drugs on the Liver. Chapter 44 - Herbal Drugs on the Liver, Liver Pathophysiology, Academic Press, 2017; 605-620. ISBN 9780128118870 [http://dx.doi.org/10.1016/B978-0-12-804274-8.00044-8].
37. Hayashi H, Sudo H. Economic importance of licorice. Plant Biotechnol J. 2009; 26(1): 101-104. ISSN 1467-7652. [https://doi.org/10.5511/PLANTBIOTECHNOLOGY.26.101].
38. Ribeiro BD, Barreto DW, Coelho M. Recovery of Saponins from Jua (Ziziphus joazeiro) by Micellar Extraction and Cloud Point Preconcentration. J Surfactants Deterg. 2013; 17(13): 553-56. ISSN 1558-9293. [http://dx.doi.org/10.1007/s11743-013-1526-5].
39. Namviriyachote N, Lipipun V, Akkhawattanangkul Y, Charoonrut P, Ritthidej GC. Development of polyurethane foam dressing containing silver and asiaticoside for healing of dermal wound. Asian J Pharm Sci. 2019; 14(1): 63-77. ISSN 1818-0876. [http://dx.doi.org/10.1016/j.ajps.2018.09.001].
40. Huang C, Huang TH, Yehet KW, Chen YLL et al. Ginsenoside Rg3 ameliorates allergic airway inflammation and oxidative stress in mice. J Ginseng Res. 2002; 45(6): 654-664. ISSN 1226-8453. [https://doi.org/10.1016/j.jgr.2021.03.002].