ARTIGO DE PESQUISA
Hexane Extracts from Piper aduncum L. in Alternative in vitro and in vivo Control of Phytopathogens of Agronomical Interest
Abstract
Even though plant extracts from Piper aduncum L. (Piperaceae) exhibit several biological activities, there are few reports in the literature of their application to the field of Agrochemistry. White mold, anthracnose and soft rot are diseases caused to plants by phytopathogens Sclerotinia sclerotiorum, Colletotrichum gloeosporioides and Rhizopus stolonifer, respectively. This study had an agrochemical approach and aimed at identifying volatile constituents in hexane extracts from P. aduncum leaves (HE-LE) and inflorescences (HE-IN) by GC-MS and GC-FID. Their in vitro and in vivo antifungal potential was also evaluated. The former was evaluated by two methodologies: the disk diffusion method (DDM) and a modified detached leaf assay (DLA). The latter was conducted in a greenhouse with 15-day-old soybean plants. Major constituents found in HE-LE were piperitone (26.0%), β-caryophyllene (11.2%) and spathulenol (12.1%) while the ones in HE-IN were piperitone (20.0%), β-caryophyllene (24.5%) and spathulenol (14.2%). All extracts under evaluation were highly efficient at inhibiting the fungus R. stolonifer. In the in vivo assay, HE-IN was highly active since it inhibited about 97% of white mold. Thus, hexane extracts from P. aduncum may be considered promising to produce a natural antifungal that aims at decreasing the use of synthetic agrochemicals.
- Keywords:
- Bioactive plant extracts.
- Organic Agrochemistry.
- Alternative control.
- Bioproducts.
Introduction
Plant extracts are natural products extracted from plants which exhibit a wide spectrum of biological activities. They may be used for fighting agricultural diseases because they are less toxic, safer to the environment, biodegradable and able to prevent crops from being attacked by pathogens[1]. Thus, they are sustainable alternatives to partially or totally substitute several synthetic agrochemicals which are considered toxic[1]. The species Piper aduncum L. stands out as a promising plant to be used in the field of Agrochemistry since it has remarkable chemical and biological properties that are directly related to its essential oils and botanic extracts[2].
P. aduncum L., whose common name is spiked pepper (pimenta-de-macaco, in Brazilian Portuguese), belongs to the Piperaceae family which is native to tropical regions and found in several biomes, such as the Amazon, Cerrado and Pampa ones[2]. Its extracts and essential oils have already exhibited antileishmanial, antibacterial, cytotoxic and antifungal activities[2].
From the biological perspective, it is believed that P. aduncum has great potential to control agricultural fungi, such as Sclerotinia sclerotiorum, Colletotrichum gloeosporioides and Rhizopus stolonifer, which cause severe diseases to plants, fruit and vegetables; an example is white mold, caused by S. Sclerotiorum to soybean plants[3]. Anthracnose is caused by C. gloeosporioides while soft rot in postharvest is caused by R. stolonifer. These fungi lead to severe damage to several crops and losses in production, storage, transport and marketing[3,4].
Considering that disease control in plants still depends much on the use of toxic synthetic fungicides, this study aimed at identifying volatile chemical constituents in hexane extracts from P. aduncum leaves (HE-LE) and inflorescences (HE-IN) by gas chromatography-mass spectrometry (GC-MS) and gas chromatography-flame ionization detection (GC-FID) (i), evaluating their in vitro antifungal activities against fungi S. sclerotiorum, C. gloeosporioides and R. stolonifer (ii) and analyzing in vivo antifungal activities of both hexane extracts in soybean crops to control white mold (S. sclerotiorum) (iii).
Material and methods
Plant material
P. aduncum L. leaves and inflorescences were collected at 9 am on February 10th, 2022, in Rio Verde, Goiás (GO), Brazil, in an environmental protection area (coordinates -17.799447S and -50.912033W) and taken to the Laboratory of Chemistry and Natural Products at the IF Goiano - Campus Rio Verde. The plant was identified by the biologist and botanist Luzia Francisca de Souza and a sample was kept at the Herbário Jataiense Professor Germano Guarim Neto (exsiccate no. HJ 7872). Access to the botanical material was approved by the Sistema Nacional de Gestão do Patrimônio Genético e do Conhecimento Tradicional Associado (SISGEN) under the code AEACDCA.
Preparation of hexane extracts
Hexane extracts were prepared with 300 g P. aduncum dry leaves and 300 g P. aduncum dry inflorescences which were selected, washed and dried by a forced air oven for 72 h. Afterwards, they were ground by a Wiley mill to reduce granulometry and placed in flat bottom balloons. Then, 1000 mL hexane PA (C6H14) was added. Both solvent and raw material kept in contact for four days at room temperature (26°C), protected from light and agitated daily. The resulting mixture of the extraction was separated by filtration with the use of filter paper, followed by solvent evaporation by a rotary evaporator under reduced pressure[5]. This process led to syrup-like hexane extracts (14 g from leaves and 6 g from inflorescences).
Identification of volatile constituents of hexane extracts
Hexane extracts were dissolved in ethyl ether and analyzed by GC-FID and GC-MS with the use of Shimadzu QP5000 Plus and GCMS2010 Plus (Shimadzu Corporation, Kyoto, Japan) systems. The temperature of the column in GC-FID was programmed to rise from 60 to 240°C at 3°C/min and was held at 240°C for 5 min; the carrier gas was H2 at flow rate of 1.0 mL/min. The equipment was set to operate in the injection mode; the injection volume was 0.1 µL (split ratio of 1:10); and injector and detector temperatures were 240 and 280°C, respectively. Relative concentrations of components were obtained by normalizing peak areas (%). Relative areas consisted of the average of triplicate GC-FID analyses. GC-MS conditions and the identification have been previously reported[6]. Identification of volatile components of hexane extracts (TABLE 1) were based on their retention indices on an Rtx-5MS (30 m X 0.25 mm; 0.250 µm) capillary column under the same operating conditions used for GC relative to a homologous series of n-alkanes (C8-C20). Structures were computer-matched with Wiley 7, NIST 08 and FFNSC 1.2 and their fragmentation patterns were compared with literature data[7].
Fungal strains
Both phytopathogenic fungi S. sclerotiorum and R. stolonifer were provided by the Laboratory of Phytopathology at the IF Goiano - Campus Rio Verde. The fungus C. gloeosporioides was isolated from contaminated papaya at the Laboratory of Phytopathology at the IF Goiano - Campus Rio Verde.
In vitro antifungal activities of HE-LE and HE-IN by the disk diffusion method
A standard solution was prepared with 4 g plant extract and 10 mL ethyl acetate to reach concentration 1 (C1 - 0.4 g/mL or 400 ppm). Eight mL of the standard solution was poured into a test tube (C1) to reach concentration 2 (C2 - 0.2 g/mL or 200ppm). Four mL ethyl acetate and 4 mL of concentration 1 (C1) were poured into another test tube and agitated to dilute completely and reach concentration 3 (C3 - 0.1 g/mL or 100 ppm). Concentration 4 (C4 - 50 ppm) resulted from the addition of 4 mL ethyl acetate and 4 mL of concentration 3, which was mixed in another test tube. Concentration 5 (C5 – 0.025 g/mL or 25 ppm) resulted from dilution of 4 mL ethyl acetate and 4 mL of concentration 4. Controls of the experiment were ethyl acetate (B - 0 ppm) and the one with no plant extract at all (T). Biological assays were carried out by a flow chamber at the Laboratory of Phytopathology at the IF Goiano - Campus Rio Verde. Petri dishes were sterilized by an autoclave and filled with potato dextrose agar (PDA) medium. Then, 0.50 μL of diluted solutions was uniformly spread on them with a Drigalski spatula. After that, 5-mm-diameter disks of PDA medium with mycelia of every fungus were placed in the center of all dishes. They were then stored in Biological Oxygen Demand (BOD) at 21ºC up to fungus growth, following the methodology described by Rezende et al.[8]. Growth was finally measured after total fungal growth on control dishes (seven days).
Percentage of inhibition of mycelial growth (IMG) was calculated by the following formula[8]:

In vitro antifungal activities of HE-LE and HE-IN by a modified detached leaf assay
The detached leaf assay followed the methodology proposed by Gabardo et al.[9] with some adaptations. Soybean leaves were detached and placed on Petri dishes with filter paper and water to keep them humid. Afterwards, leaves were inoculated with 5-mm-diameter disks of S. sclerotiorum culture grown in PDA medium for four days and were incubated in a germination chamber at 22 ± 3ºC for 24 h under a 12-h photoperiod in a BOD incubator. After the incubation period, soybean leaves were sprinkled with their respective experimental treatments. Solutions of hexane extracts were prepared with distilled water, ethyl acetate and tween 80 to reduce toxicity in soybean leaves. Solutions that had the most active concentrations were HE-LE (C1 - 0.4 g/mL and C2 - 0.2 g/mL) and HE-IN (C1 - 0.4 g/mL and C2 - 0.2 g/mL). Besides, both treatments were used: control (B - 0 g/mL) and control with no treatment (T). Right after hexane extracts were sprinkled, Petri dishes were incubated again at 22 ± 3ºC under a 12-h photoperiod in BOD. The first evaluation started after 12 h of incubation and was carried out until total growth of the control with no treatment (T). The leaf lesion was measured by a digital pachymeter.
Outlining and statistics of in vitro assays
The experiment had a completely randomized design in a factorial scheme which consisted of two types of extracts from P. aduncum (leaves and inflorescences), three species of fungi (S. sclerotiorum, C. gloeosporioides and R. stolonifer) and seven treatments composed of different concentrations of hexane extracts. Concentrations resulted from dilution of plant extract in ethyl acetate PA in a serial dilution scheme at the following concentrations: 0.4; 0.2; 0.1; 0.05; 0.025 g/mL, the solvent ethyl acetate (white) and the control with no extract with five replicates per treatment, thus, totaling 210 experimental units (2x3x7x5=210). Data on percentages of IMG were subject to the normality test which showed the normal distribution of parameters under evaluation. Regarding descriptive statistics, data were subject to the analysis of variance (ANOVA), the Tukey's test (p<0.05) and the R Core Team 8.0 software program. Results were expressed as tables.
In vivo antifungal assay in soybean plants
Glycine max plants, super-early cycle, were grown in 2-kg plastic bags filled with substrate – sand and NPK (4-30-16) – and were kept in a greenhouse at room temperature and manual irrigation for 15 days under a 12-h photoperiod. The methodology proposed by Garcia et al.[10] was applied. Fifteen-day-old soybean plants were taken to the Laboratory of Chemistry and Natural Products at the IF Goiano - Campus Rio Verde to have their leaves inoculated with the fungus. Inoculation took place when 7-mm-diameter disks with the fungus grown in PDA medium for four days were inserted into the adaxial sides of leaves. After inoculation, plants were kept at 22ºC ± 3ºC at 90% humidity for 24 h under a 12-h photoperiod to enable early fungal growth. After 24 h, soybean plants were treated with the solutions of the most active extracts at the same concentrations employed in the detached leaf assay. The most active concentrations were HE-LE (C1 - 0.4 g/mL and C2 - 0.2 g/mL) and HE-IN (C1 - 0.4 g/mL and C2 - 0.2 g/mL). In addition, both the control (B - 0 g/mL) and the control with no treatment (T) were evaluated. Right after the application of sprinkle treatments with the use of a manual sprinkler, leaves were kept at 22ºC ± 3ºC at 90% humidity under a 12-h photoperiod. Areas of leaf lesions were measured by a digital pachymeter 24 h after application of treatments up to total growth of S. sclerotiorum on leaves of plants in the negative control.
Outlining and statistical analysis of in vivo assays
In vivo experiments had a completely randomized design in a factorial scheme that consisted of six treatments: control, control with no treatment and the four most active concentrations of HE-LE and HE-IN, with five replicates per treatment, totaling 60 experimental units (2x6x5=60). Data on percentages of IMG were subject to the normality test (Shapiro-Wilk)[11].
Results and Discussion
Volatile constituents of HE-LE and HE-IN were identified by GC-FID and GC-MS. Total percentages of constituents identified in HE-LE and HE-IN were 98.2% and 97.1%, respectively. Major constituents found in HE-LE were piperitone (26.0%), β-caryophyllene (11.2%) and spathulenol (12.1%) (TABLE 1) while the ones identified in HE-IN were piperitone (20.0%), β-caryophyllene (24.5%) and spathulenol (14.2%) (TABLE 1).
Compound | RT (min) | RIexp | RIlit | % RA | |
HE-LE | HE-IN | ||||
Terpinen-4-ol | 20.57 | 1180 | 1179 | 10.0 | 10.5 |
Piperitone | 24.31 | 1252 | 1254 | 26.0 | 20.0 |
α-cubebene | 33.57 | 1350 | 1351 | 0.9 | 0.2 |
β-elemene | 34.59 | 1373 | 1375 | 1.9 | 2.3 |
Isoledene | 34.76 | 1376 | 1377 | 0.5 | — |
β-cubebene | 35.33 | 1389 | 1390 | 6.5 | — |
β-caryophyllene | 36.43 | 1416 | 1418 | 11.2 | 24.5 |
γ-elemene | 36.78 | 1425 | 1423 | 5.0 | — |
Aromadendrene | 37.10 | 1438 | 1439 | 2.1 | 1.0 |
α-selinene | 37.71 | 1449 | 1451 | — | 1.6 |
α-humulene | 37.94 | 1455 | 1455 | 0.1 | — |
Alloaromadendrene | 38.12 | 1459 | 1460 | — | 2.0 |
β-Cadinene | 38.58 | 1471 | 1472 | 0.1 | 5.2 |
γ-muurolene | 38.76 | 1475 | 1476 | 1.3 | 1.0 |
β-selinene | 38.88 | 1483 | 1485 | 6.8 | — |
Valencene | 39.38 | 1491 | 1491 | 4.5 | 3.4 |
Bicyclogermacrene | 39.56 | 1493 | 1493 | 2.9 | 3.5 |
α-Cadinene | 41.15 | 1537 | 1538 | 1.0 | — |
Germacrene B | 41.95 | 1559 | 1560 | 3.1 | 0.7 |
Spathulenol | 42.48 | 1574 | 1575 | 12.1 | 14.2 |
Isoaromadendrene oxide | 42.71 | 1578 | 1579 | — | 2.0 |
Caryophyllene oxide | 42.89 | 1583 | 1581 | 0.8 | 0.5 |
Viridiflorol | 43.16 | 1589 | 1590 | 0.6 | — |
Humulene epoxide | 43.93 | 1610 | 1609 | — | 0.9 |
Isospathulenol | 44.23 | 1630 | 1631 | 0.8 | — |
α-muurolol | 45.19 | 1645 | 1645 | — | 1.1 |
Aromadendrene oxide | 45.91 | 1667 | 1668 | — | 1.8 |
Alloaromadendrene oxide | 47.03 | 1696 | 1697 | — | 0.7 |
Total | 98.2 | 97.1 | |||
RT = Retention time; RIexp = Retention index relative to n-alkanes (C8–C20) on the Rtx-5MS column; RIlit = Kovats retention index (values from the literature[7]). %RA = Relative abundance. -: not detected. Bold numbers mean that volatile constituents were considered major constituents of hexane extracts. |
Results of this study disagree with the ones found by Lucena et al.[12], whose GC-MS analysis showed that hexane extract from P. aduncum leaves was mainly composed of apiole (90.7%). It should be highlighted that apiole, a phenylpropanoid, was identified neither in HE-LE nor in HE-IN from this species found in the Cerrado Goiano Biome. A study conducted by Santos and collaborators[13] reports that major constituents of hexane extract from P. aduncum were caryophyllene, α-calacorene, γ-elemene, cis-γ-elemene, germacrene D, linalool, linalool oxide, nerolidol, β-elemene, δ-cadinene, α-caryophyllene and falcarinol. The fact that some chemical constituents are found in some extracts from P. aduncum but not in others may be explained by activities of biotic and abiotic factors, such as soil, seasonality, harvest period, material preparation and part of plants under study[14].
Different concentrations of HE-LE against S. sclerotiorum, C. gloeosporioides and R. stolonifer exhibited low coefficients of variation in statistical analyses. Treatments with the highest concentrations – 0.4 and 0.2 g/mL in treatments C1 and C2, respectively – did not differ statistically from each other and exhibited the highest mean values of inhibition against the three fungi under evaluation. R. stolonifer exhibited the highest inhibition since mean values were about 80% at the highest doses (C1 and C2). S. sclerotiorum exhibited lower values of inhibition in all treatments; they did not reach 50% of mycelial inhibition. C. gloeosporioides had its best result at concentration C1 since inhibition reached 80.73%. Finally, both the control (B) and the control with no treatment (T) were statistically equal since there was no fungal inhibition (TABLE 2).
Fungi | % inhibition | ||||||
C1 | C2 | C3 | C4 | C5 | B | T | |
SSC | 48.02 b | 43.53 c | 17.88 c | 8.18 a | 0.0 b | 0.0 a | 0.0 a |
RST | 94.42 a | 93.78 a | 73.87 a | 74.03 a | 61.64 a | 0.0 a | 0.0 a |
CGL | 80.73 c | 67.78 b | 63.78 b | 17.28 b | 0.0 b | 0.0 a | 0.0 a |
*Means followed by the same letter in a column do not differ statistically from each other by the Tukey's test at 5% probability (p=0.005). SSC: S. sclerotiorum. RST: R. stolonifer. CGL: C. gloeosporioides. |
In assays with HE-IN, concentrations 0.4 and 0.2 g/mL, treatments C1 and C2, did not differ statistically from each other and exhibited high mean values of fungal inhibition. HE-IN was able to inhibit 100% of S. sclerotiorum growth (TABLE 3). Against C. gloeosporioides, the best result of inhibition – 80.73% – was reached at C1. This percentage was also reached by HE-LE. It should be emphasized that HE-IN was more efficient against R. stolonifer since inhibition was about 60% even at the lowest concentration, i. e., 0.05 g/mL (C4) (TABLE 3). It should also be mentioned that, in both control (B) and control with no treatment (T), all fungi exhibited total development, a fact that reassures that ethyl acetate is not fungitoxic and did not affect results of in vitro assays.
Fungi | % inhibition | ||||||
C1 | C2 | C3 | C4 | C5 | B | T | |
SSC | 100 a | 100 a | 53.65 a | 46.62 b | 36.62 a | 0.0 a | 0.0 a |
RST | 89.60 b | 83.73 b | 73.04 a | 69.40 a | 0.0 b | 0.0 a | 0.0 a |
CGL | 80.73 c | 67.56 c | 63.78 b | 17.28 c | 0.0 b | 0.0 a | 0.0 a |
*Means followed by the same letter in a column do not differ statistically from each other by the Tukey's test at 5% probability (p=0.005). SSC: S. sclerotiorum. RST: R. stolonifer. CGL: C. gloeosporioides. |
Results of this study of HE-LE and HE-IN agree with and complement the study carried out by Valadares and collaborators[4], who showed that essential oils from P. aduncum leaves and inflorescences were highly active against S. sclerotiorum. The fact that hexane extracts and essential oils are liposoluble enables their permeability through cell membranes, leading to cell death due to loss of ions and cell material and, consequently, preventing fungal development from taking place[15]. Another reason that may explain the fact that HE-IN exhibits the best antifungal activity is its high concentration of the chemical constituent β-caryophyllene (24.5%), a powerful in vitro antifungal against phytopathogens[16]. β-caryophyllene is a hydrophobic molecule, which presents low molecular weight; these properties facilitate the permeability in the cellular membrane of the fungi, being able to be involved in the mechanism of action of inhibition of microbial growth[16]. Besides, HE-IN exhibits the sesquiterpene spathulenol in higher concentration (14.2%). Spathulenol may also reinforce that activity exhibited by HE-IN is better than the one shown by HE-LE since the chemical constituent has promising antimicrobial potential[17]. Furthermore, piperitone was able to favor mycelial inhibition of different fungi according to the literature[18].
In the detached leaf assay applied to soybean plants, with the use of HE-LE and HE-IN, treatments with the highest doses of extracts (C1 and C2) significantly inhibited fungal growth and leaf necrosis. This analysis was carried out five days after inoculation of disks with the chosen fungus, i. e., S. sclerotiorum (FIGURE 1). HE-LE exhibited higher viscosity and lower effectiveness and versatility than HE-IN throughout application. Treatments with no extracts – control (B) and control with no treatment (T) – exhibited high level of damage to leaf tissues as the result of fast fungal contamination; fungal mycelia may be easily seen in FIGURE 1. Results of the detached leaf assay are shown in TABLE 4.
% inhibition | |
S. sclerotiorum | |
HE-LE [C1] | 43.86 c |
HE-LE [C2] | 51.01 b |
HE-IN [C1] | 99.07 a |
HE-IN [C2] | 98.96 a |
B | 0.0 d |
T | 0.0 d |
* Means followed by the same letter in a column do not differ statistically from each other by the Tukey's test at 5% probability (p=0.005). [C1] and [C2] are the highest concentrations used in the assays. |
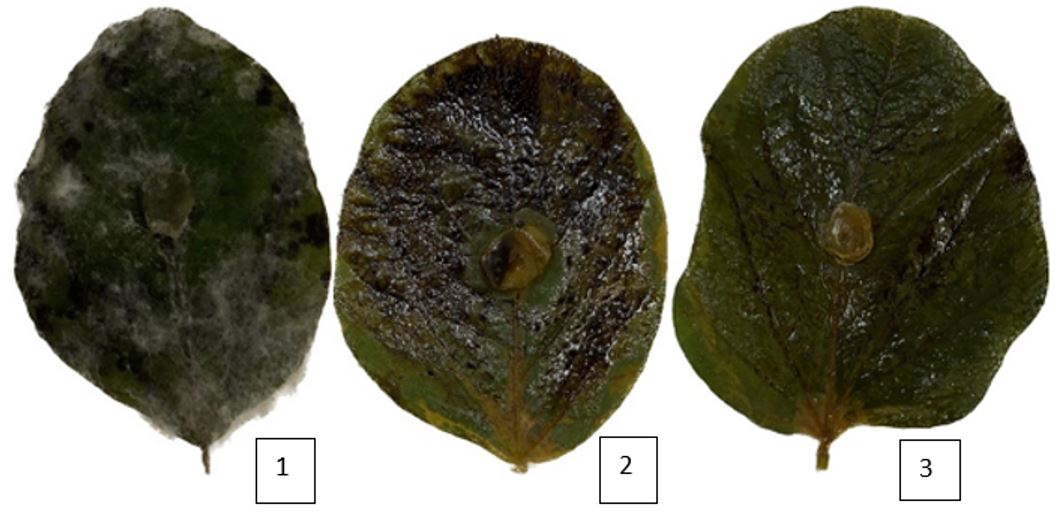
HE-LE exhibited low percentages of inhibition of mycelial growth, i. e., 43% (C1) and 51% (C2), while HE-IN had relevant results at the same concentrations – around 98% - and showed its high control over S. sclerotiorum. This data reveals the promising potential of HE-IN as a fungicide. It should be noted that detached leaf assays are very promising to determine efficiency of extracts to control diseases since conditions in which pathogens colonize plants, such as temperature and humidity, are excellent and favor maximum expression of diseases in plant tissues. This fact was shown by Fonseca et al.[19] who stated that diseases caused by Rhizoctonia solani and Sclerotium rolfsii were satisfactorily controlled in detached leaves since extracts under evaluation were as active as the commercial fungicide used by their study.
In vivo assays in soybean showed little difference in percentages of fungal inhibition by comparison with the detached leaf assay. Application of HE-IN to soybean plants led to effective inhibition of S. sclerotiorum growth (around 97%; TABLE 5). Besides, neither necrosis nor sick areas were formed (FIGURE 2) shows that the control plant was completely consumed by white mold whereas the plant treated with HE-IN at concentration C1 kept healthy major structures. HE-LE exhibited poor inhibitory activity, whose values were 40.42% (C1) and 42.33% (C2) (Table 5). Once again, control (B) showed that ethyl acetate, which was used for diluting the extracts, did not interfere with fungal growth.
% inhibition | |
S. sclerotiorum | |
HE-LE [C1] | 40.42 b |
HE-LE [C2] | 42.33 b |
HE-IN [C1] | 98.84 a |
HE-IN [C2] | 97.54 a |
B | 0.0 c |
T | 0.0 c |
Means followed by the same letter in a column do not differ statistically from each other by the Tukey's test at 5% probability (p=0.005). [C1] and [C2] are the highest concentrations used in the assays. |
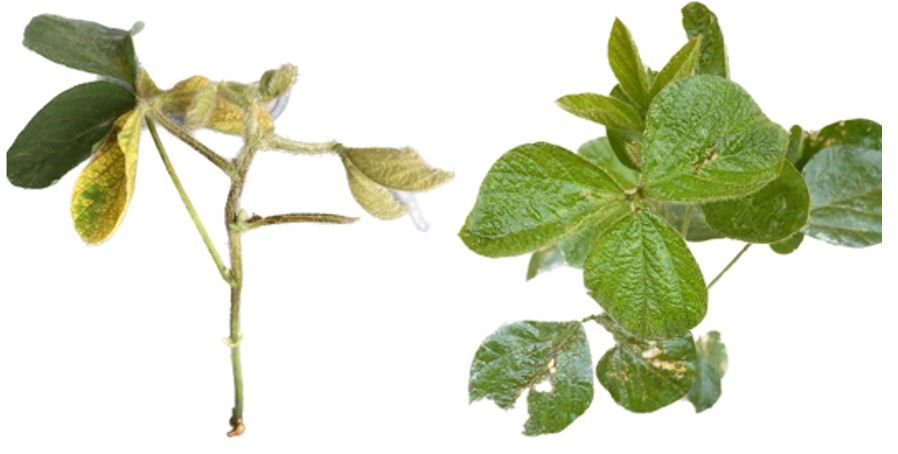
In vitro and in vivo assays have proven that HE-IN has promising antifungicidal activity against the fungus that causes white mold to soybean. It is implied that viscosity and syrup-like consistency of HE-LE may have interfered negatively with results of the assays. Besides, variation in fungal inhibition resulting from concentrations of hexane extracts showed the relation of dependence between concentrations of natural products and inhibition of agricultural fungi. Concerning plant extracts, the literature reports that the higher the concentration of bioactive secondary metabolites and the synergy among these compounds, the more potentialized the fungitoxic property[20].
On the other hand, it should be highlighted that in vivo assays with plants are subject to biological responses given by the plants themselves against phytopathogens. Plants exhibit mechanisms of defense when they are attacked by pathogens and start to respond immediately after the attack by producing specific compounds as defense and resistance, thus, leading to abortion of injured or contaminated structures[21]. However, studies of plant extracts that aim at controlling fungi that attack plants are important in the field of Agrochemistry since they enable family and organic farmers to use alternative, sustainable and less toxic solutions[20].
Conclusion
The major constituents found in HE-LE were piperitone, β-caryophyllene and spathulenol while the ones in HE-IN were the same. In the conditions of the biological experiments, the conclusion is that HE-IN exhibits the best inhibitory activity over mycelial growth of fungi under evaluation while HE-LE showed low activity in in vitro and in vivo assays. HE-IN was highly active in all assays, but HE-LE was not. In sum, this study contributed positively to further studies of the species P. aduncum so as to promote the development of natural agrochemicals based on bioactive compounds from plants.
Funding
The authors would like to thank the IFGOIANO - Campus Rio Verde, FAPEMA, FAPEG, CNPq and CAPES for their financial support.
Conflicts of Interest
The authors declare no conflict of interest.
Collaborators
Funding acquisition and supervision: CCF; MLDM.
Conceptualization, investigation, methodology: VPM; IRRS; MNX; AEMC; JGB; MSA.
Writing, original draft and Writing, review & editing: MLDM.
Referências
1. Brito NM, Nascimento LC. Potencial fungitóxico de extratos vegetais sobre Curvularia eragrostidis (P. Henn.) Meyer in vitro. Rev Bras Pl Med. 2015; 17(2): 230-38. ISSN: 1516-0572. [acesso em: 10 jan 2023]. Disponível em: [https://doi.org/10.1590/1983-084X/10_057].
2. Morais VP, Cabral FV, Fernandes CC, Miranda MLD. Brief review on Piper aduncum L., its bioactive metabolites and its potential to develop bioproducts. Braz Arch Biol Technol. 2023; 66(1): e23220314. ISSN: 1678-4324. [acesso em: 10 jan. 2023]. Disponível em: [https://doi.org/10.1590/1678-4324-2023220314].
3. Toigo SEM, Fernandes CC, Miranda MLD. Promising antifungal activity of two varieties of Capsicum chinense against Sclerotinia sclerotiorum, Rhizopus stolonifer and Colletotrichum goleosporoides. Food Sci Technol. 2022; 42(1): e52722. ISSN: 0101-2061. [acesso em: 10 jan. 2023]. Disponível em: [https://doi.org/10.1590/fst.52722].
4. Valadares ACF, Fernandes CC, Filho JGO, Deus IPB, Lima TM, Silva EAJ, et al. Incorporation of essential oils from Piper aduncum into films made from arrowroot starch: effects on their physicochemical properties and antifungal activity. Quim Nova. 2020; 43(6): 729-37. ISSN: 0100-4042. [acesso em: 10 jan. 2023]. Disponível em: [https://doi.org/10.21577/0100-4042.20170530].
5. Ribeiro MMJ, Silva KMM, Palavecino LA, Pinto LC, Ferreira BLA, Lobão AQ, et al. Anatomical, Histochemical and biological studies of Clusia grandiflora Splitg. (Clusiaceae). Braz Arch Biol Technol. 2020; 63: e20190674. ISSN: 1678-4324. [acesso em: 10 jan. 2023]. Disponível em: [https://doi.org/10.1590/1678-4324-2020190674].
6. Cabral FV, Fernandes CC, Dias ALB, Ribeiro AB, Squarisi IS, Tavares DC, et al. Hexane extract from Tradescantia pallida (Rose) D. R. Hunt (Commelinaceae): its volatile constituents and in vitro antifungal and cytotoxic activities. Braz Arch Biol Technol. 2022; 65: e22210621. ISSN: 1678-4324. [acesso em: 22 jan. 2023]. Disponível em: [https://doi.org/10.1590/1678-4324-2022210621].
7. Adams RP. Identification of essential oil components by gas chromatography/mass spectrometry. Carol Stream, Illinois, USA: Allured Publishing Corporation, 2007.
8. Rezende JL, Fernandes CC, Costa OAM, Santos LS, Vicente Neto F, Sperandio EM, et al. Antifungal potential of essential oils from two varieties of Citrus sinensis (lima orange and bahia navel orange) in postharvest control of Rhizopus stolonifer (Ehrenb.: Fr.) Vuill. Food Sci Technol. 2020; 40(Suppl. 2): 405-09. ISSN: 0101-2061. [acesso em: 10 jan. 2023]. Disponível em: [https://doi.org/10.1590/fst.30519].
9. Gabardo G, Pria MD, Silva HL, Harms MG. Método da folha destacada para avaliação da indução de resistência de produtos alternativos a Phakopsora pachyrhizi, Sclerotinia sclerotiorum e Rhizoctonia solani em soja. Braz J Develop. 2020; 6(7): 43847-62. ISSN: 2525-8761. [acesso em: 24 mar. 2023]. Disponível em: [https://doi.org/10.35587/brj.ed.0000607].
10. Garcia RÁ, Meyer MC, Ávila KAGB, Cunha MG. Métodos de inoculação de Sclerotinia sclerotiorum para triagem de cultivares de soja resistentes ao mofo branco. Pesq Agropec Bras. 2015; 50(8): 726-29. ISSN: 0100-204X. [acesso em: 10 jan. 2023]. Disponível em: [https://doi.org/10.1590/S0100-204X2015000800011].
11. Neto SC, Silva JF, Souza MC, Silva HF, Silva EC, Nascimento LC. Controle de Colletotrichum spp. em maracujazeiro amarelo com elicitores de resistência. Rev Bras Meio Amb. 2023; 11(1): 157-64. ISSN: 2595-4431. [acesso em: jan. 2023]. Disponível em: [https://www.revistabrasileirademeioambiente.com/index.php/RVBMA/article/view/1410].
12. Lucena DC, Bertholdo-Vargas LR, Silva WC, Machado AF, Lopes TS, Moura S, et al. Biological activity of Piper aduncum extracts on Anticarsia gemmatalis (Hubner) (Lepdoptera: Erebdae) and Spodoptera frugiperda (J.E. Smith) (Lepidoptera: Noctuidae). An Acad Bras Ciênc. 2017; 89(3): 1869-79. ISSN: 0001-3765. [acesso em: 10 jan. 2023]. Disponível em: [https://doi.org/10.1590/0001-3765201720170194].
13. Santos ML, Magalhães CF, Rosa MB, Santos DA, Brasileiro BG, Carvalho LM, et al. Antifungal activity of extracts from Piper aduncum leaves prepared by different solvents and extraction techniques against dermatophytes Trichophyton rubrum and Trichophyton interdigitale. Braz J Microbiol. 2013; 44(4): 1275-78. ISSN: 1678-4405. [acesso em: 24 mar. 2023]. Disponível em: [https://doi.org/10.1590/S1517-83822013000400035].
14. Souza AO, Bessa DHRF, Fernandes CC, Pereira PS, Martins CHG, Miranda MLD. Phytochemical screening of extracts from Spiranthera odoratissima A. St.-Hil. (Rutaceae) leaves and their in vitro antioxidant and anti-Listeria monocytogenes activities. Acta Scient Biol Sci. 2020; 42: e51881. ISSN: 1807-863X. [acesso em: 10 jan 2023]. Disponível em: [https://doi.org/10.4025/actascibiolsci.v42i1.51881].
15. Toigo SEM, Fernandes CC, Miranda MLD. Promising antifungal activity of two varieties of Capsicum chinense against Sclerotinia sclerotiorum, Rhizopus stolonifer and Colletotrichum goleosporoides. Food Sci Technol.2022; 42: e52722. ISSN: 0101-2061. [acesso em: 10 jan. 2022]. Disponível em: [https://doi.org/10.1590/fst.52722].
16. Sobrinho ACN, Morais SM, Souza EB, Albuquerque MRJR, Santos HS, Cavalcante CSP, et al. Antifungal and antioxidant activities of Vernonia chalybaea Mart. ex DC. Essential oil and their major constituent β-caryophyllene. Braz Arch Biol Technol. 2020; 63: e20190177. [acesso em: 10 jan. 2023]. Disponível em: [https://doi.org/10.1590/1678-4324-2020190177].
17. Gazella LN, Glamoclija J, Sokovic M, Gonçalves JE, Linde GA, Colauto NB et al. Antimicrobial Activity of Essential Oil of Baccharis dracunculifolia DC (Asteraceae) Aerial Parts at Flowering Period. Front Pl Sci. 2019; 10: 27. ISSN: 1664-462X. [acesso em: 10 jan 2023]. Disponível em: [https://doi.org/10.3389/fpls.2019.00027].
18. Wei S, Xu Q, Pei S, Lv Y, Lei Y, Zhang S, Zhai H, Hu Y. Unraveling the antifungal and anti-aflatoxin B1 mechanisms of piperitone on Aspergillus flavus. Food Microbiol. 2024; 123: 104588. [acesso em: 19 set. 2024]. Disponível em: [https://doi.org/10.1016/j.fm.2024.104588].
19. Fonseca AS, Fernandes CF, Júnior JRV, Rocha RB, Freire TC, Sangi SC et al. Extratos vegetais do gênero Capsicum com potencial atividade antifúngica contra Rhizoctonia solani e Sclerotium rolfsii. Rev Ibero Amer Ciênc Amb. 2020; 11(1): 89-98. ISSN: 2179-6858. [acesso em: 10 jan. 2023]. Disponível em: [https://doi.org/10.6008/CBPC2179-6858.2020.001.0010].
20. Vilamil ES, Demosthenes LCR, Caniato FF, Bentes JLS, Neto PQC. Efeito do extrato vegetal de Piper callosum sp. In vitro sobre Colletrotrichum sp. agente etiológico da antracnose do pimentão. Braz J Develop. 2021; 7(3): 27655-67. ISSN: 2525-8761. [acesso em: 10 jan. 2023]. Disponível em: [https://doi.org/10.34117/bjdv7n3-462].
21. Stangarlin JR, Kuhn OJ, Toledo MV, Portz RL, Schwan-Estrada KRF, Pascholati SF. A defesa vegetal contra fitopatógenos. Scientia Agr Paranaensis. 2011; 10(1): 18-46. ISSN: 1983-1471. [acesso em: 10 jan. 2023]. Disponível em: [https://doi.org/10.18188/sap.v10i1.5268].