Artigo de Pesquisa
Cryptomeria japonica (L. F.) D. Don as source of shikimic acid
Abstract
Oseltamivir phosphate (OSP), marketed under the brand name of Tamiflu®, is a potent inhibitor of viral neuraminidase, a glycoside hydrolase enzyme essential for viral replication. OSP is also important to control influenza virus (H1N1) and is produced mainly from shikimic acid (SA) extracted from plants. The main source of SA is from seeds of I. verum. Alternatives to meet the worldwide demand are necessary. One of this is Cryptomeria japonica. Inthis study, we found a content of 56.36 mg.kg-1 of SA in dried biomass for C. japonica, while for I.verum, the traditional source, was 96.57 mg.kg-1. Due to the fact of C. japonica is a tree, exploited for commercial production, it can be a renewable and reliable source SA for OSP production.
- Keywords:
- Influenza.
- Tamiflu.
- Oseltamivir phosphate.
- Neuraminidase.
Introduction
There are four main influenza virus species: A, B, C and D. Type A viruses are known to infect a wide variety of birds and mammals, while the other species have more restricted host ranges[1]. Occasionally the world suffers influenza pandemics like Spanish flu in 1918, Asian flu in 1957 and Hong Kong flu in 1968[2].The first influenza pandemic A (H1N1) of the 21st century lasted 14 months from June 2009 to August 2010[2]. Avian influenza H5N1, originated in poultry in Hong Kong in 1997, is also a major concern worldwide with a lethality rate over 50%[3].
Shikimic acid (SA), [(3R,4S,5R)-3,4,5-trihydroxycyclohex-1-ene-1-carboxylic acid)] is a major intermediate in the biosynthesis of essential amino acids important for plant survival[4]. A large number of plant metabolites depend on SA via the shikimate pathway, found in many tissues of a variety of plants, depending on the age and growth stage of the plants. SA content is higher in stages where the metabolic process rates are high as in intensive growth according to the organ of plants[4].
Neuramidases are glycoside hydrolase enzymes that cleave the glycosidic bonds of neuraminic acid essential for viral replication[5]. Oseltamivir phosphate (OSP) is a potent inhibitor of viral neuraminidase developed in 1996 by a Californian-based pharmaceutical company[6] and launched in 1999 by the pharmaceutical company Roche, marketed under the brand name Tamiflu®, used against various strains of influenza virus, including H1N1 and H5N1[7].
Shikimic acid (FIGURE 1) precursor of OSP is the main chiral starting compound, in a complex synthesis process, involving 11 phases for its production[4]. SA supply has been a problem for the increased demand of OSP[4].
Although many scientists have been working to develop synthetic processes to produce SA[8] such as genetically engineering microbes of E. coli strain[9], the most important source of SA is from plants[10].
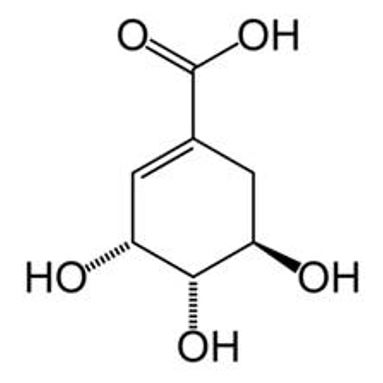
In terms of source supply, the majority of world´s production of SA is from seeds of Illicium verum, a shrub native from China, popularly known as Chinese star anise, and Illiciumm anizatum, native from Japan[11,12]. Cultivation of this specie is very difficult because it reaches seed bearing stage only after six years of sowing with seeds containing about 2-7% of SA[11,12].
As result, alternatives source of SA is very desirable to meet the worldwide demand of Tamiflu®[13]. In a conservative estimate, Bradley[14] states that 520,000 kg of SA are needed for producing Tamiflu® in the event of a pandemic affecting 400 million people, compared to a current annual production of 100,000 kg of the acid.
Cryptomeria japonica, Cupressaceae, gymnosperm, is a native conifer from Japan and China, widely distributed in rainy regions, cold winters and moderately warm summers. According Kratz[15] this conifer is a large tree species, light wood and reddish color. In Brazil, it has a rapid growth, good adaptation to the climate and soil of the southern region of the country and a good response to growing technologies. Besides its potential as a wood, it is a potential source of essential oils for use in the pharmaceutical industry[16].
Because of those properties mentioned before, we selected C. japonica in this study to find an alternate renewable source of this acid comparing to the usual source of Star anise seeds (I. verum).
Material and methods
Plant material
A prior study was conducted to explore other alternative sources of SA from Cupressaceae family like Thuja spp. In this case SA content varyed from 0.12 to 0.52% (w/w), what led us to choose C. japonica for further studies. Since the purpose of this research was to explore the potential of steady supply of a source of SA, fully grown mature branches of adult plants of C. japonica, produced by vegetative propagation from cuttings were collected in Colombo, PR, Brazil, at the Forestry Research Center of Embrapa Florestas (Brazilian Agricultural Research Corporation) (25o 19'18.20" S and 49o 9'34.47" W). No pesticides including glyphosate were applied during any time on the plants. The branches were harvested on December 2017. Since this was a study to evaluate the potential of the plant to produce SA we chose only one season to develop the protocol. Star anise seeds, I. verum, used as reference were purchased locally.
Extraction of SA
Branches with needles from C. japonica were harvested and processed at the Laboratory, located at Jaguariúna, SP, Brazil, and kept under refrigeration. Measurement of SA content was conducted by a modified method according to Matallo et al.[17]. The whole branches were oven dried at 40oC for 72 hours, grounded with a knife mill (Tecnal, Brazil), sealed in a polystyrene bag and stored in a -18oC until analyses. Aliquots of 400 mg of dry matter with ten replications each one, were mixed with 10 mL of Milli-Q water acidified to pH 3 with formic acid, kept in microwave for 50 seconds at 100 W (Panasonic NN-S62B) reaching temperature of 50ºC (± 3.0ºC), cooled for 5 minutes, filtered through Whatman Nº 1 filter paper (0.22 µm) (Millex-GV, Millipore) for quantification by Liquid Chromatography coupled to a Mass Spectrometer (LC-MS).
Chemicals and reagents
The solvents utilized as mobile phase was methanol Lichrosolv, HPLC grade (Merck) and ammonium acetate (Merck) and SA (99.6%) standard, purchased from Sigma-Aldrich.
LC-MS analysis of SA
SA detection and confirmation were performed by Liquid Chromatography (UFLC – Shimadzu) coupled to an Ion Trap Mass Spectrometry (Bruker Daltonics - Model amaZonTM X), with Electrospray (ESI) as ionization source. Chromatographic conditions: UFLC: Auto sampler: SIL – 20 AC; Column oven: CTO-20; Communications Bus Module: CBM-20A; High pressure pumps: LC-20AT; Degasser: DGU-20As, Column: Lichrosorb - RP-18–100Å, 250 x 4,60mm – 5 µm – Phenomenex; Security Guard cartridges: – Fusion – RP – 4 x 3.0mm – Phenomenex; injection volume - 20 µL; Flow rate: 0.800 mL.min-1; Oven temperature: 40°C; mobile phase: Isocratic mode: 10% Methanol HPLC grade: 90% ammonium acetate; run time: 7 minutes, mass spectrometer in negative mode. Capillary voltage 4500 V. Nitrogen was used as nebulization gas at 320°C at flow rate of 10 L.min-1 and pressure of 60 psi with Helium as collision gas. Retention time of SA was 3.2 minutes. A t test was performed to compare the amount of SA in both extracts (*P<0.05). In order to evaluate the method precision, SA samples were analyzed in ten replicates.
Results and Discussion
SA standard was used and its mass spectra had a [M-1]- ion at m/z 172.6 according to C7H10O5 and the ions m/z 154.6, the highest intensity, 143.7, 110.8 and 92.9. 154.6 ion was chosen for quantification and the others for confirmation. The identification of the peak corresponding to the SA in the samples was achieved comparing the mass spectra and the retention time of the sample and the SA standard (FIGURE 2).
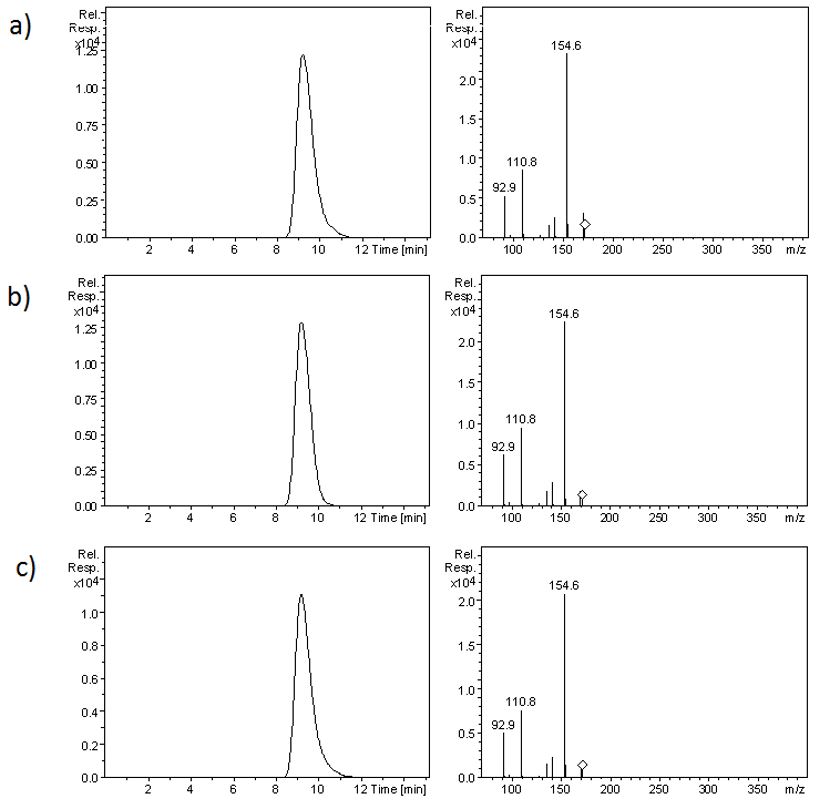
The amount of SA found in C. japonica and I.verum (branches and seeds respectively) were determined by Microwave Assisted Extraction (MWAE)[17] using acidified water, as described in the experimental part. The quantifications resulted in 56.36 (± 3.31) mg.kg-1 and the CV of 5.9% for the dried biomass for C. japonica and 96.57 (± 6.24) mg.kg-1 e CV of 6.5% for I. verum, representing 5.64 (± 0.33) and 9.68 (± 0.62) percentage respectively (w/w). The t-test showed a significant difference between the levels of SA in both extracts (p=1,15.10-8) (FIGURE 3).
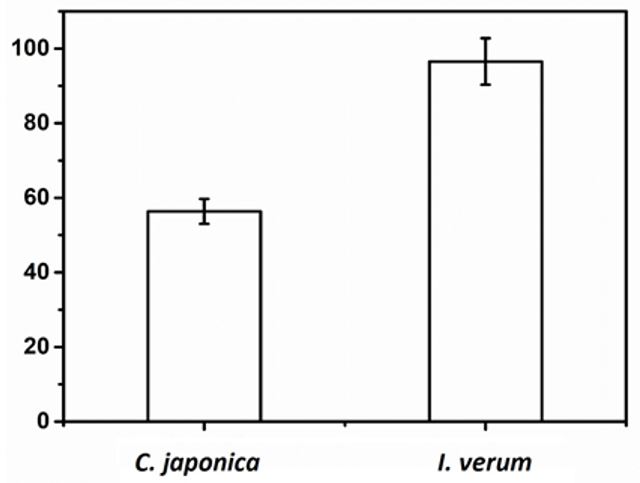
Different species yielded SA at various levels ranged from 0.001% to 24.5%, the highest content reported for I. religiosum [18]. In our results, the SA content found of 5.64 % on dry basis in C. Japonica is on the range compared to other species related by Bochkov et al.[18].
SA accumulates in gymnosperms and woody dicots. Sui[19], found concentrations of 0.82 to 1.6% in the wet and dried biomass of pine needles. Raghavendra et al.[13] screened 210 trees species and found detectable levels of shikimic acid, with six species accumulating greater than 1% by dry weight. Enrich et al.[20] found levels ranging from 2.4 to 3.7%, w/w from the seeds of Liquidambar styraciflua.
We believe that the fast growth of C. japonica, the amount that we found and the easy adaptability to the edapho-climatic conditions in the southern of Brazil associated with the good response to the forestry technologies can make this species as an alternate source of SA[15].
The results shows that branches of C. japonica have reasonably concentration of SA when compared with I. verum corresponding to 58.36% of that, representing a renewable source of SA. C. japonica typically yields 45 m³.ha-1 from crop biomass on a per-hectare basis and their woody have a low density about 0.40 g.cm-3 [21], which could potentially produce 1,000 kg of SA/ha (gross weight).
Conclusion
Besides the use as wood, C. japonica can be exploited for commercial production of SA and can alleviate the problem of SA scarcity. Optimization of SA from C. japonica isolation at scale is still required to confirm the commercial viability of this plant as precursor of Oseltamivir phosphate in the pharmaceutical and cosmetic industry. Also more data on seasonal variation studies of SA content is necessary.
Acknowledgments
Marley Mendonça Tavares, Embrapa Meio Ambiente, by carrying out the chemical analyses. Rosana Higa, Embrapa Floresta for helping on plant material harvesting. Project funded by CNPq (Conselho Nacional de Desenvolvimento Científico e Tecnológico).
References
1. Lycett SJ, Duchatel F, Digard P. A brief history of bird flu. Philos Trans Royal Soc B. 2019; 374(1775). ISSN 0962-8436. [CrossRef].
2. Martirosyan L, Paget WJ, Jorgensen P, Brown CS, Meerhoff TJ, Pereyaslov D et al. The community impact of the 2009 influenza pandemic in the WHO European Region: a comparison with historical seasonal data from 28 countries. BMC Infect Dis [Internet]. 2012 Feb 12; 12: 36. Available from: [CrossRef] [PubMed].
3. Farina V, Brown JD. Tamiflu: The supply problem. Angew Chem. 2006; 45(44): 7330-4. ISSN 1433-7851. [CrossRef] [PubMed].
4. Abrecht S, Federspiel MC, Estermann H, Fischer R, Karpf M, Mair HJ et al. The synthetic-technical development of oseltamivir phosphate tamifluTM: A race against time. Chimia Inter J Chem. 2007; 61(3): 93-9. ISSN 0009-4293. [CrossRef].
5. Forleo-Neto E, Halker E, Santos VJ, Paiva TM, Toniolo-Neto J. Influenza. Rev Soc Bras Med Trop. 2003; 36(2): 267-74. ISSN 0037-8682. [CrossRef] [PubMed].
6. Kim CU, Lew W, Williams MA, Liu HT, Zhang LJ, Swaminathan S et al. Influenza neuraminidase inhibitors possessing a novel hydrophobic interaction in the enzyme active site: Design, synthesis, and structural analysis of carbocyclic sialic acid analogues with potent anti-influenza activity. J Am Chem Soc. 1997; 119(4): 681-90. ISSN 0002-7863. [CrossRef] [PubMed].
7. Kramer M, Bongaerts J, Bovenberg R, Kremer S, Muller U, Orf S et al. Metabolic engineering for microbial production of shikimic acid. Metab Eng. 2003; 5(4): 277-83. ISSN 1096-7176. [CrossRef].
8. Borah JC. Shikimic acid: a highly prospective molecule in pharmaceutical industry. Curr Sci. 2015; 109(9): 1672-9. ISSN 0011-3891. [CrossRef].
9. Díaz Quiroz DC, Carmona SB, Bolívar F, A E. Current perspectives on applications of shikimic and aminoshikimic acids in pharmaceutical chemistry. Res Rep Med Chem. 2014; 4: 35-46. [CrossRef].
10. Cardoso SF, Lopes LMX, Nascimento IR. Eichhornia crassipes: an advantageous source of shikimic acid. Rev Bras Farmacogn. 2014; 24(4): 439-42. ISSN 0102-695X. [CrossRef].
11. Payne R, Edmonds M. Isolation of shikimic acid from star aniseed. J Chem Educ. 2005; 82(4): 599-600. ISSN 0021-9584. [CrossRef].
12. Sadaka M, Garcia A. Extraction of shikimic and quinic acids. Chem Eng Commun. 1999; 173(1): 91-102. ISSN 0098-6445. [CrossRef].
13. Raghavendra TR, Vaidyanathan P, Swathi HK, Ramesha BT, Ravikanth G, Ganeshaiah KN et al. Prospecting for alternate sources of shikimic acid, a precursor of Tamiflu, a bird-flu drug. Curr Sci. 2009; 96(6): 771-2. ISSN 0011-3891. [Link].
14. Bradley D. Star role for bacteria in controlling flu pandemic? Nat Rev Drug Discov. 2005; 4: 945. [CrossRef].
15. Kratz D, Wendling I, Brondani G.E. Concentrações de ácido indolbutírico no enraizamento de Cryptomeria japonica. J Biotec Biodivers. 2014; 2(3): 14-21. ISSN 2179-4804.
16. Santos CBd, Longhi SJ, Hoppe JM, Moscovich FA. Efeito do volume de tubetes e tipo de substratos na qualidade de mudas de Cryptomeria japonica (L.F.) D. Don. Cien Flore. 2000; 10(2): 1-15. [CrossRef].
17. Matallo MB, Almeida SDB, Cerdeira AL, Franco DA, Blanco FMG, Menezes PTC et al. Microwave-assisted solvent extraction and analysis of shikimic acid from plant tissues. Pl Dan. 2009; 27(spe): 987-94. ISSN 0100-8358. [CrossRef].
18. Bochkov DV, Sysolyatin SV, Kalashnikov AI, Surmacheva IA. Shikimic acid: review of its analytical, isolation, and purification techniques from plant and microbial sources. J Chem Biol. 2012; 5: 5-17. [CrossRef].
19. Sui R. Separation of shikimic acid from pine needles. Chem Eng Technol. 2008; 31(3): 469-73. ISSN 0930-7516. [CrossRef].
20. Enrich LB, Scheuermann ML, Mohadjer A, Matthias KR, Eller CF, Newman MS et al. Liquidambar styraciflua: a renewable source of shikimic acid. Tetrahedron Lett. 2008; 49(16): 2503-5. ISSN 0040-4039. [CrossRef].
21. Shimizu JY, Maiochi RA. Criptoméria como espécie alternativa para produção de madeira no Paraná. Pesqui Florest Bras. 2007; 5(54): 63-70. ISSN 1983-2605. [Link].